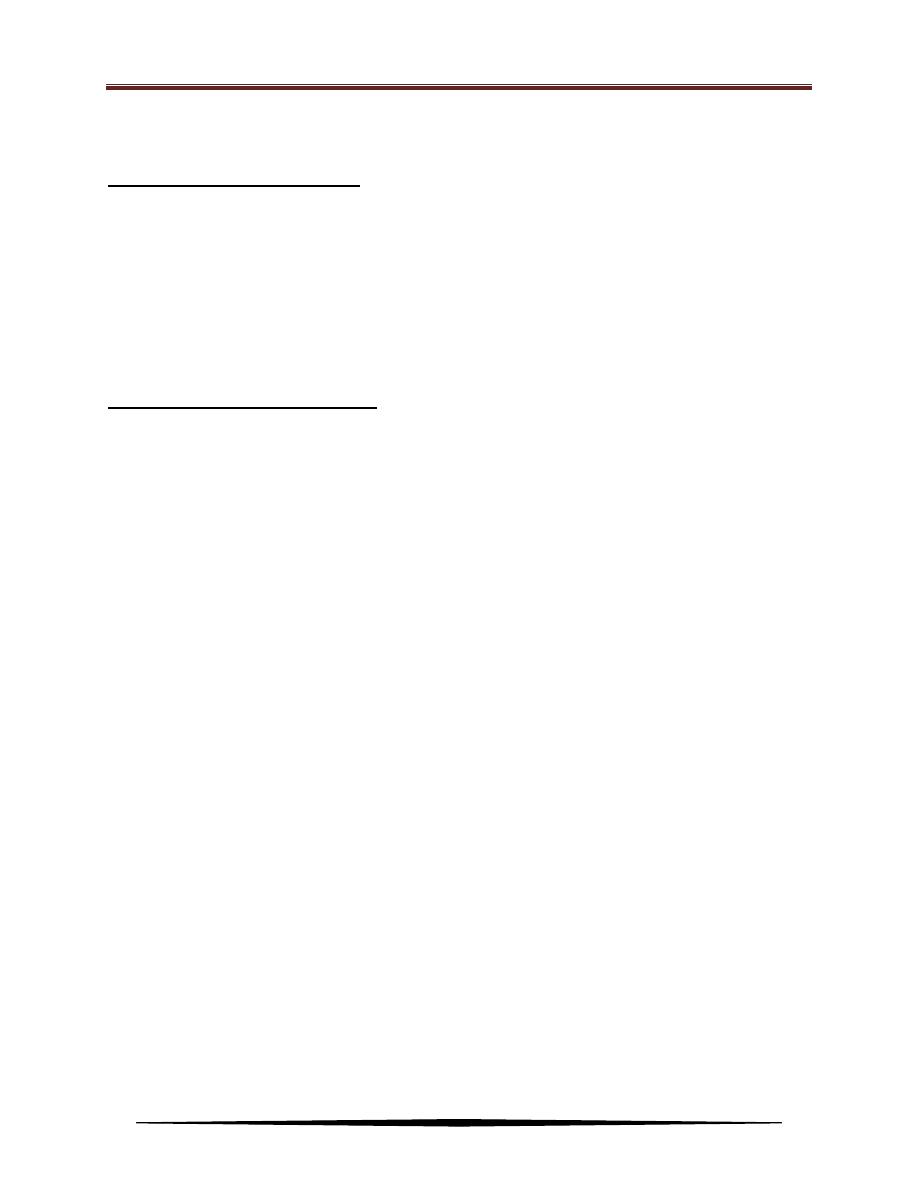
Chemistry for 1st class/Physics
Dr. Alaa Hussein Jalil
1
States of Matter
Classifications of Matter
Matter is anything that occupies space and has mass, and chemistry is the
study of matter and the changes it undergoes. There are two principal ways of
classifying matter: by its physical state as a solid, liquid, or gas and by its
chemical constitution as an element, compound, or mixture.
Solids, Liquids, and Gases
Commonly, a given kind of matter exists in different physical forms under
different conditions. Water, for example, exists as ice (solid water), as liquid water,
and as steam (gaseous water). The main identifying characteristic of solids is their
rigidity: they tend to maintain their shapes when subjected to outside forces.
Liquids and gases, however, are fluids; that is, they flow easily and change their
shapes in response to slight outside forces.
What distinguishes a gas from a liquid is the characteristic of compressibility
(and its opposite, expansibility). A gas is easily compressible, whereas a liquid is
not. These two characteristics, rigidity (or fluidity) and compressibility (or
expansibility), can be used to frame definitions of the three common states of
matter:
Solid the form of matter characterized by rigidity; a solid is relatively
incompressible and has fixed shape and volume.
Liquid the form of matter that is a relatively incompressible fluid; a liquid
has a fixed volume but no fixed shape.
Gas the form of matter that is an easily compressible fluid; a given quantity
of gas will fit into a container of almost any size and shape.
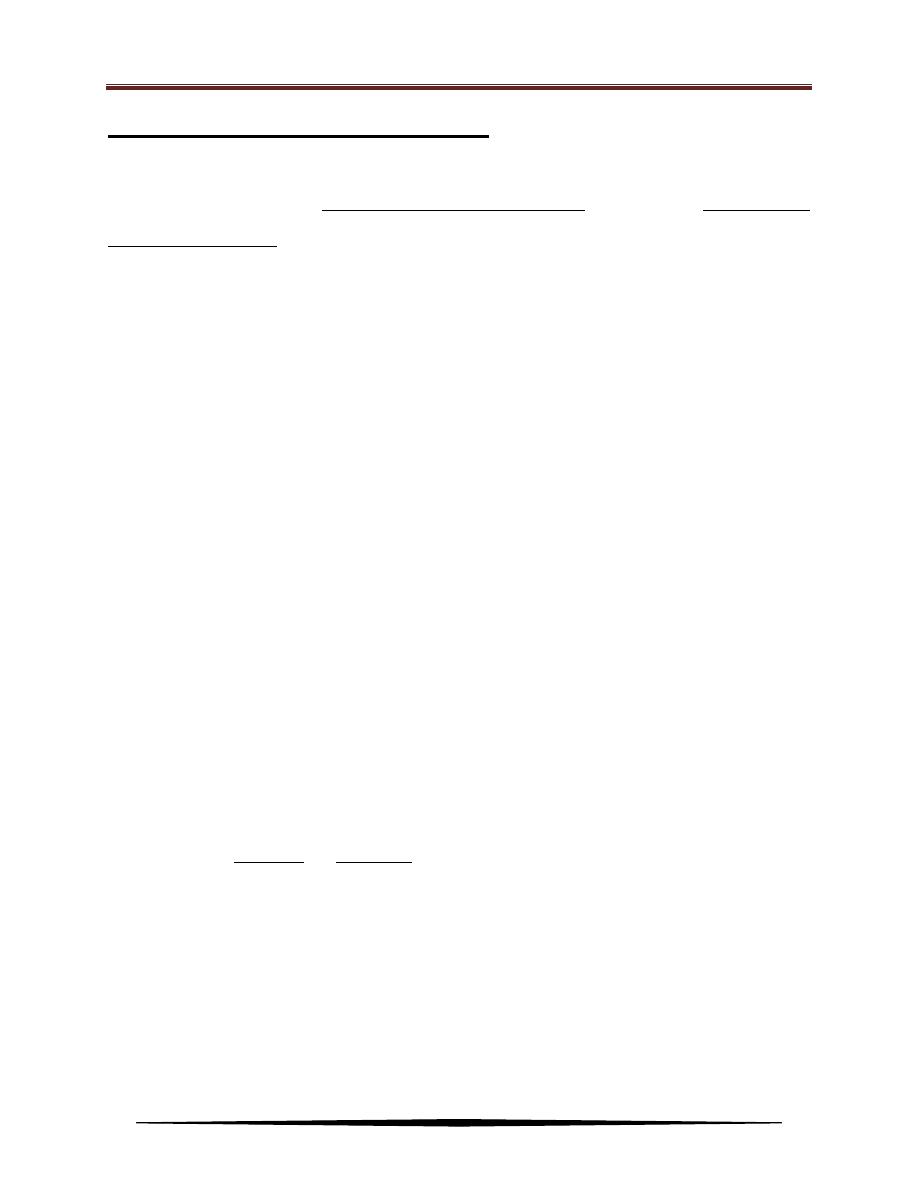
Chemistry for 1st class/Physics
Dr. Alaa Hussein Jalil
2
Elements, Compounds, and Mixtures
To understand how matter is classified by its chemical constitution, we must
first distinguish between physical and chemical changes and between physical and
chemical properties. A physical change is a change in the form of matter but not
in its chemical identity. Changes of physical state are examples of physical
changes. The process of dissolving one material in another is a further example of
a physical change. For instance, sodium chloride (table salt) dissolves in water.
The result is a clear liquid, like pure water, though many of its other characteristics
are different from those of pure water. The water and sodium chloride in this liquid
retain their chemical identities and can be separated by some method that depends
on physical changes. Distillation is one way to separate the sodium chloride and
water components of this liquid.
A chemical change, or chemical reaction, is a change in which one or
more kinds of matter are transformed into a new kind of matter or several new
kinds of matter. The rusting of iron, during which iron combines with oxygen in
the air to form a new material called rust, is a chemical change. The original
materials (iron and oxygen) combine chemically and cannot be separated by any
physical means. To recover the iron and oxygen from rust requires a chemical
change or a series of chemical changes.
A material can be characterized or identified by its various properties, which
may be either physical or chemical. A physical property is a characteristic that
can be observed for a material without changing its chemical identity. Examples
are physical state (solid, liquid, or gas), melting point, and color. A chemical
property is a characteristic of a material involving its chemical change. A
chemical property of iron is its ability to react with oxygen to produce rust.
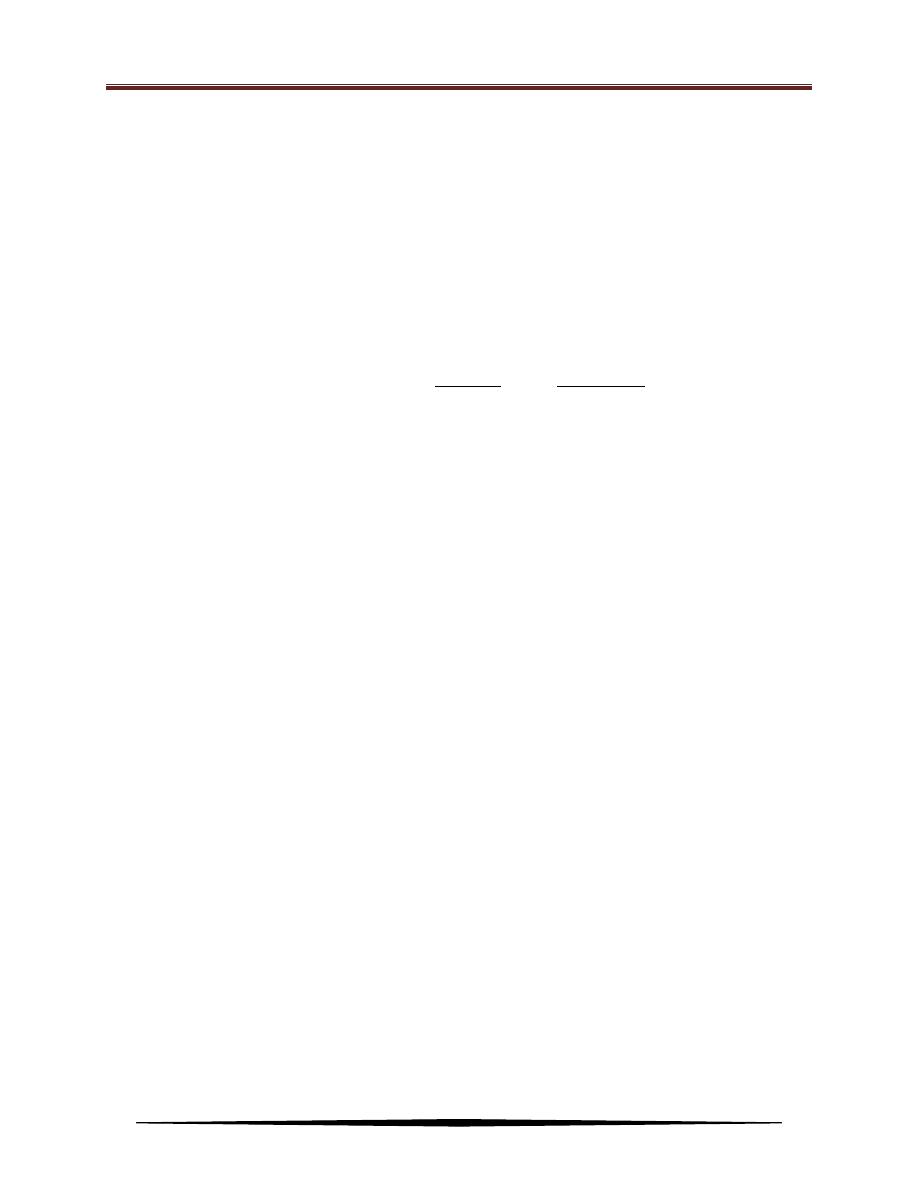
Chemistry for 1st class/Physics
Dr. Alaa Hussein Jalil
3
The various materials around us are either substances or mixtures of
substances. A substance is a kind of matter that cannot be separated into other
kinds of matter by any physical process. For example, it is possible to separate the
sodium chloride from the water by the physical process of distillation, but sodium
chloride is itself a substance and cannot be separated by physical processes into
new materials. Similarly, pure water is a substance. A substance always has the
same characteristic properties.
A substance can be either an element or a compound. An element is a
substance that cannot be separated into simpler substances by chemical means.
Today 116 elements are known. Most substances are compounds. A compound is
a substance composed of two or more elements chemically combined. Hydrogen
gas, for example, burns in oxygen gas to form water, a compound whose properties
are distinctly different from those of the starting materials. Water is made up of
two parts of hydrogen and one part of oxygen. This composition does not change.
Most of the materials around us are mixtures. A mixture is a material that
can be separated by physical means into two or more substances. Mixtures are
classified into two types. A heterogeneous mixture is a mixture that consists of
physically distinct parts, each with different properties. A homogeneous mixture
(also known as a solution) is a mixture that is uniform in its properties throughout
given samples. Any mixture, whether homogeneous or heterogeneous, can be
created and then separated by physical means into pure components without
changing the identities of the components. Thus, sugar can be recovered from a
water solution by heating the solution and evaporating it to dryness. Condensing
the water vapor will give us back the water component. To separate the iron-sand
mixture, we can use a magnet to remove the iron filings from the sand, because
sand is not attracted to the magnet. After separation, the components of the mixture
will have the same composition and properties as they did to start with.
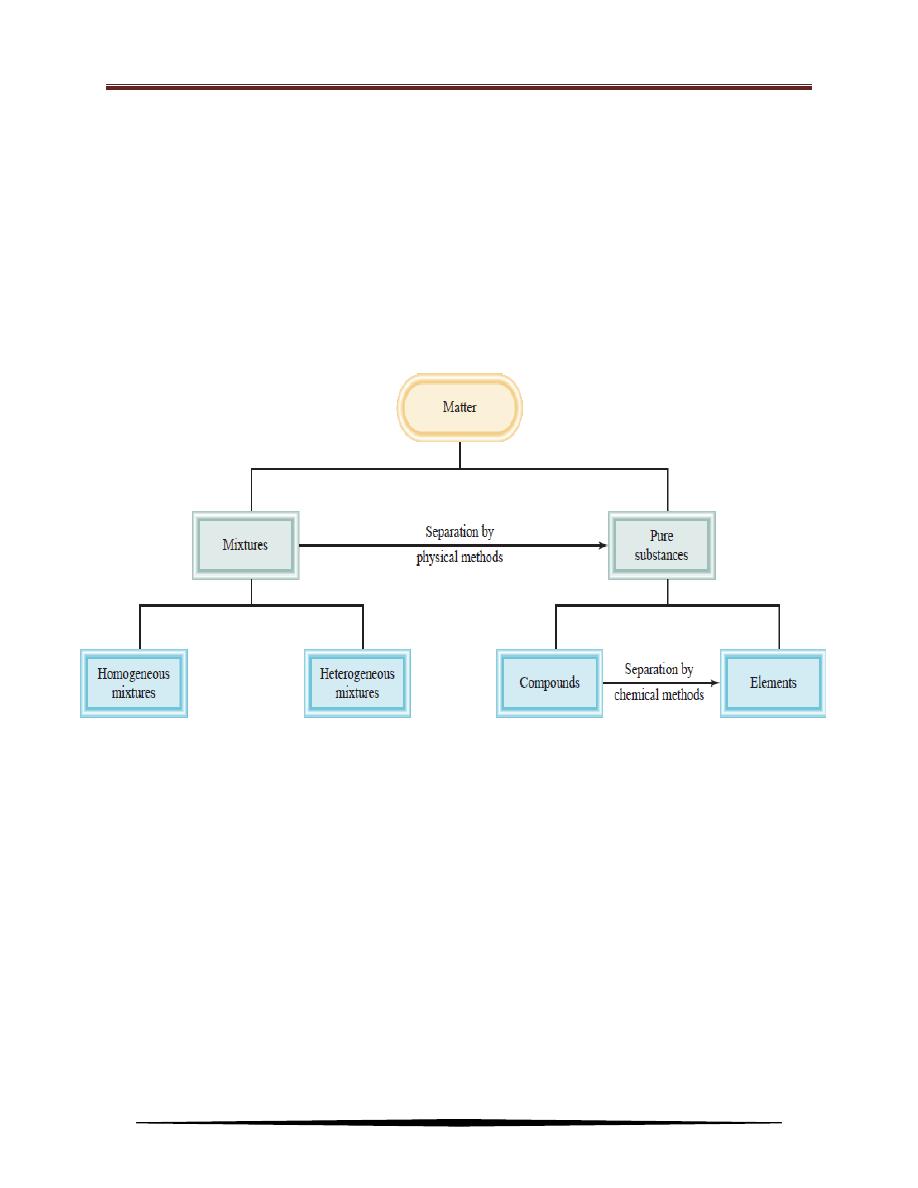
Chemistry for 1st class/Physics
Dr. Alaa Hussein Jalil
4
The relationships among elements, compounds, and other categories of
matter are summarized in the Figure below. Materials are either substances or
mixtures. Substances can be mixed by physical processes, and other physical
processes can be used to separate the mixtures into substances. Substances are
either elements or compounds. Elements may react chemically to yield
compounds, and compounds may be decomposed by chemical reactions into
elements.
All measurable properties of matter fall into two categories: extensive
properties and intensive properties. The measured value of an extensive property
depends on how much matter is being considered. Mass, length, and volume are
extensive properties. More matter means more mass. Values of the same extensive
property can be added together. For example, two copper pennies have a combined
mass that is the sum of the masses of each penny, and the total volume occupied by
the water in two beakers is the sum of the volumes of the water in each of the
beakers.
Classification of matter
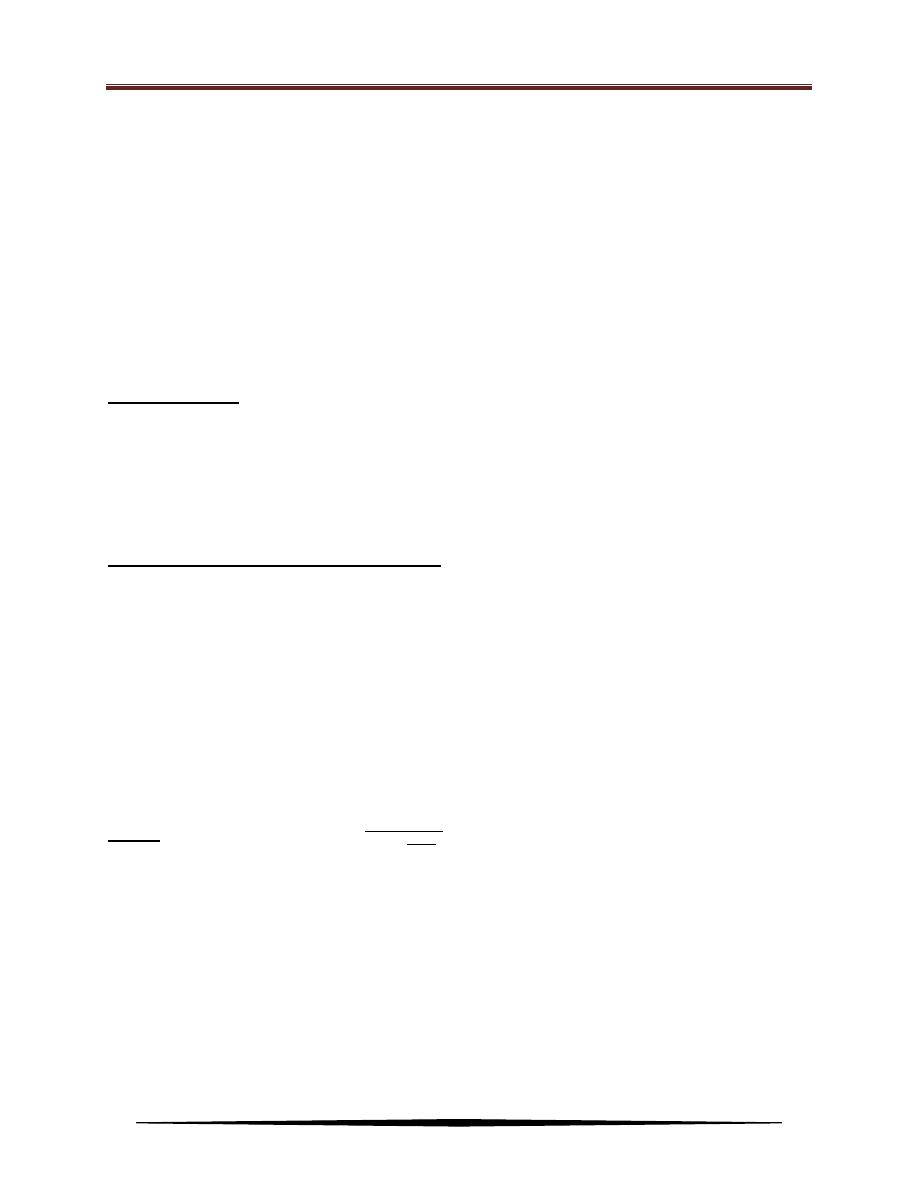
Chemistry for 1st class/Physics
Dr. Alaa Hussein Jalil
5
The measured value of an intensive property does not depend on the amount
of matter being considered. Temperature is an intensive property. Suppose that we
have two beakers of water at the same temperature. If we combine them to make a
single quantity of water in a larger beaker, the temperature of the larger amount of
water will be the same as it was in two separate beakers. Unlike mass and volume,
temperature and other intensive properties such as melting point, boiling point, and
density are not additive.
Atomic mass
The atomic mass or atomic mass unit (amu) of an element is the average
atomic mass for the naturally occurring element, expressed in atomic mass units
(atomic mass is sometimes referred to as atomic weight).
Molar mass and Molecular mass
The molar mass of a substance is the mass of one mole of the substance.
The molecular mass (MM) of a substance is the sum of the atomic masses of all
the atoms in a molecule of the substance. For all substances, the molar mass in
grams per mole is numerically equal to the molecular mass in atomic mass units.
Ethanol, for example, whose molecular formula is C
2
H
6
O has a molecular mass of
46.1 amu and a molar mass of 46.1 g/mol.
Mole
[
]
A mole (symbol mol) is defined as the quantity of a given substance that
contains as many molecules or formula units as the number of atoms in exactly 12
g of carbon-12. One mole of ethanol, for example, contains the same number of
ethanol molecules as there are carbon atoms in 12 g of carbon-12. The number of
atoms in a 12-g sample of carbon-12 is called Avogadro’s number (symbol N
A
)
(this number give the value 6.02 x10
23
).
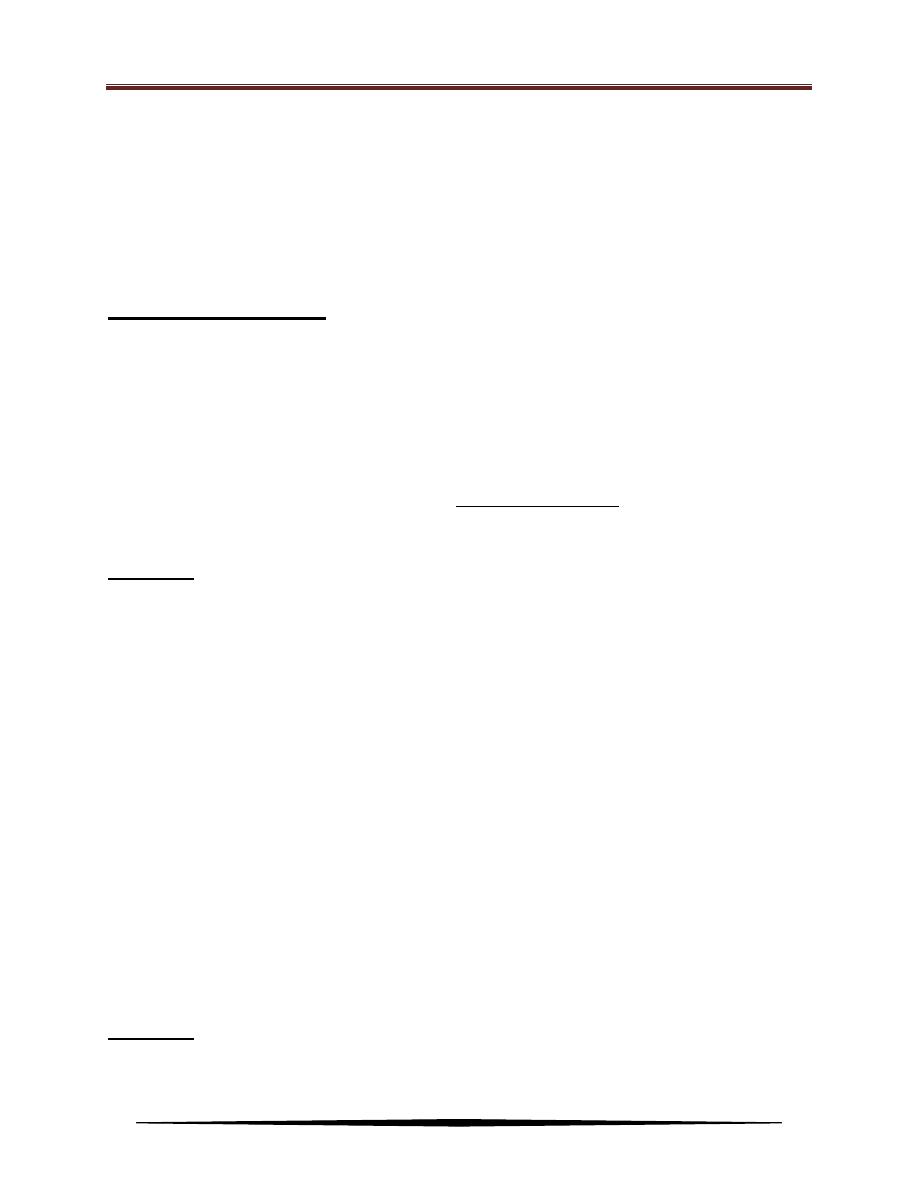
Chemistry for 1st class/Physics
Dr. Alaa Hussein Jalil
6
A mole of a substance contains Avogadro’s number (6.02 x 10
23
) of
molecules. When using the term mole, it is important to specify the formula of the
unit to avoid any misunderstanding. For example, a mole of oxygen atoms (with
the formula O) contains 6.02 x10
23
O atoms. A mole of oxygen molecules
(formula O
2
) contains 6.02 x10
23
O
2
molecules—that is, 2 x (6.02 x 10
23
) O atoms.
Molar Concentration
When we dissolve a substance in a liquid, we call the substance the solute
and the liquid the solvent. The general term concentration refers to the quantity of
solute in a standard quantity of solution. Molar concentration, or molarity (M), is
defined as the moles of solute dissolved in one liter (cubic decimeter) of solution.
Example: A sample of NaNO
3
weighing 0.38 g is placed in a 50.0 mL volumetric
flask. The flask is then filled with water to the mark on the neck,
dissolving the solid. What is the molarity of the resulting solution?
Qualitatively, we say that a solution is dilute when the solute concentration
is low and concentrated when the solute concentration is high. Usually these
terms are used in a comparative sense and do not refer to a specific concentration.
We say that one solution is more dilute, or less concentrated, than another. The
relationship between the molarity of the solution before dilution (the initial
molarity) and that after dilution (the final molarity) is:
M
i
x V
i
= M
f
x V
f
where M
i
for the initial molar concentration, V
i
for the initial volume of solution,
M
f
(the final molar concentration) and V
f
(the final volume).
Example: You are given a solution of 14.8 M NH
3
. How many milliliters of this
solution do you require to give 100.0 mL of 1.00 M NH
3
when diluted.
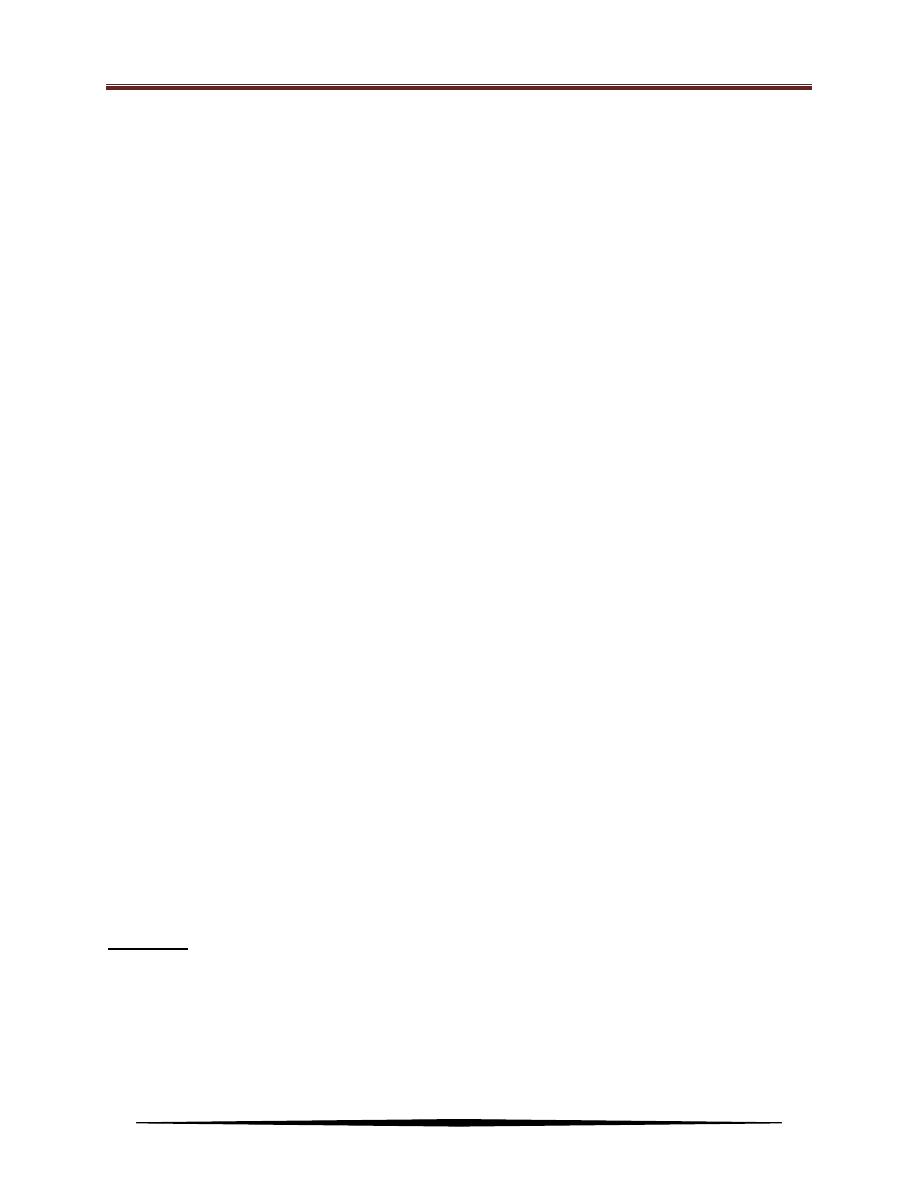
Chemistry for 1st class/Physics
Dr. Alaa Hussein Jalil
7
GASES
Particles in a gas are far apart, fast-moving, and are not organized in any
particular way. Unlike the particles in solids and liquids, the atoms and molecules
in gases are not particularly attracted to each other.
In gases, the intermolecular forces that hold molecules together in liquids
and some solids are still present, but gas molecules overcome these intermolecular
forces with speed. Individual gas molecules are always on the move; they have a
lot of energy that keeps them moving constantly.
As a result, within a gas, the atoms or molecules pass each other regularly
and interact only for a brief moment of time. This short bit of time is not enough
for intermolecular forces to take hold and act. As a result, the atoms and molecules
in a gas continue on their own way.
This lack of force holding atoms or molecules together is what determines
the most unique properties of gases. A gas will expand in all directions to fill any
space, and will spread to take on the shape of its container. But it is not the same as
a liquid. There are no other forces, with the exception of gravity, to hold a gas in
place.
In this state of matter, it’s not so much the forces acting between atoms or
molecules that are important. Instead, three other factors determine the movements
of atoms or molecules in a gas: temperature, pressure, and volume. Chemists relate
these three factors in a series of gas laws.
Volume
Volume is length (m) cubed, so its SI-derived unit is the cubic meter (m
3
).
Generally, however, chemists work with much smaller volumes, such as the cubic
centimeter (cm
3
) and the cubic decimeter (dm
3
):
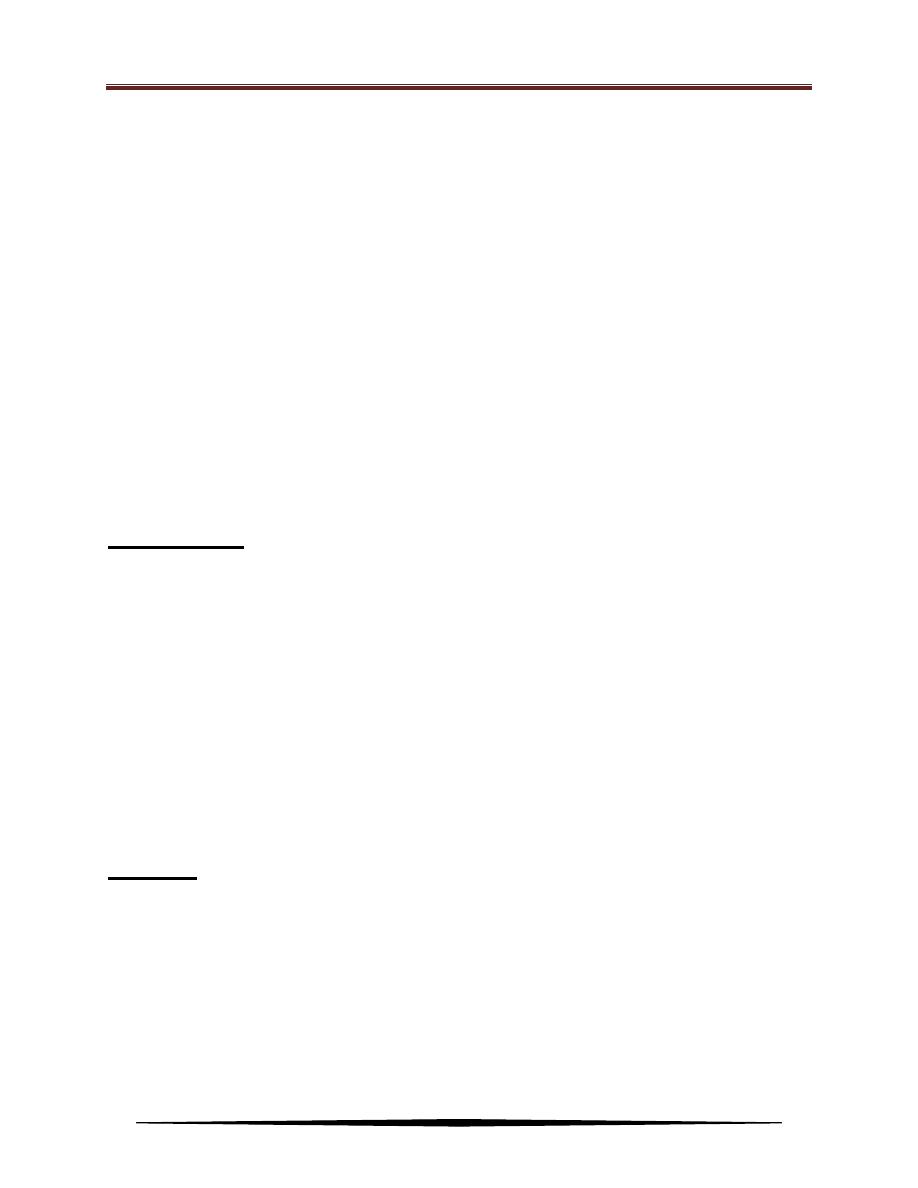
Chemistry for 1st class/Physics
Dr. Alaa Hussein Jalil
8
1 cm
3
= 1 x10
-6
m
3
1 dm
3
= 1 x10
-3
m
3
Another common, non-SI unit of volume is the liter (L). A liter is the volume
occupied by one cubic decimeter. Chemists generally use L and mL for liquid
volume. One liter is equal to 1000 milliliters (mL) or 1000 cubic centimeters:
1 L = 1000 mL
1 L = 1000 cm
3
1 L = 1 dm
3
and one milliliter is equal to one cubic centimeter:
1 mL = 1 cm
3
Temperature
Temperature is not just about how hot or cold something feels.
Temperature is actually a measurement of the average kinetic energy in a
material. Kinetic energy refers to the energy of motion. Particles within matter are
always in motion. The faster the particles within a sample of matter move, the
higher its kinetic energy, or temperature.
The slower the particles move, the lower its kinetic energy, or temperature.
Gases at warm temperatures have fast-moving particles.
Pressure
Pressure is one of the most readily measurable properties of a gas. It may be
expressed in many different units. To understand how we measure the pressure of a
gas, it is helpful to know how the units of measurement are derived.
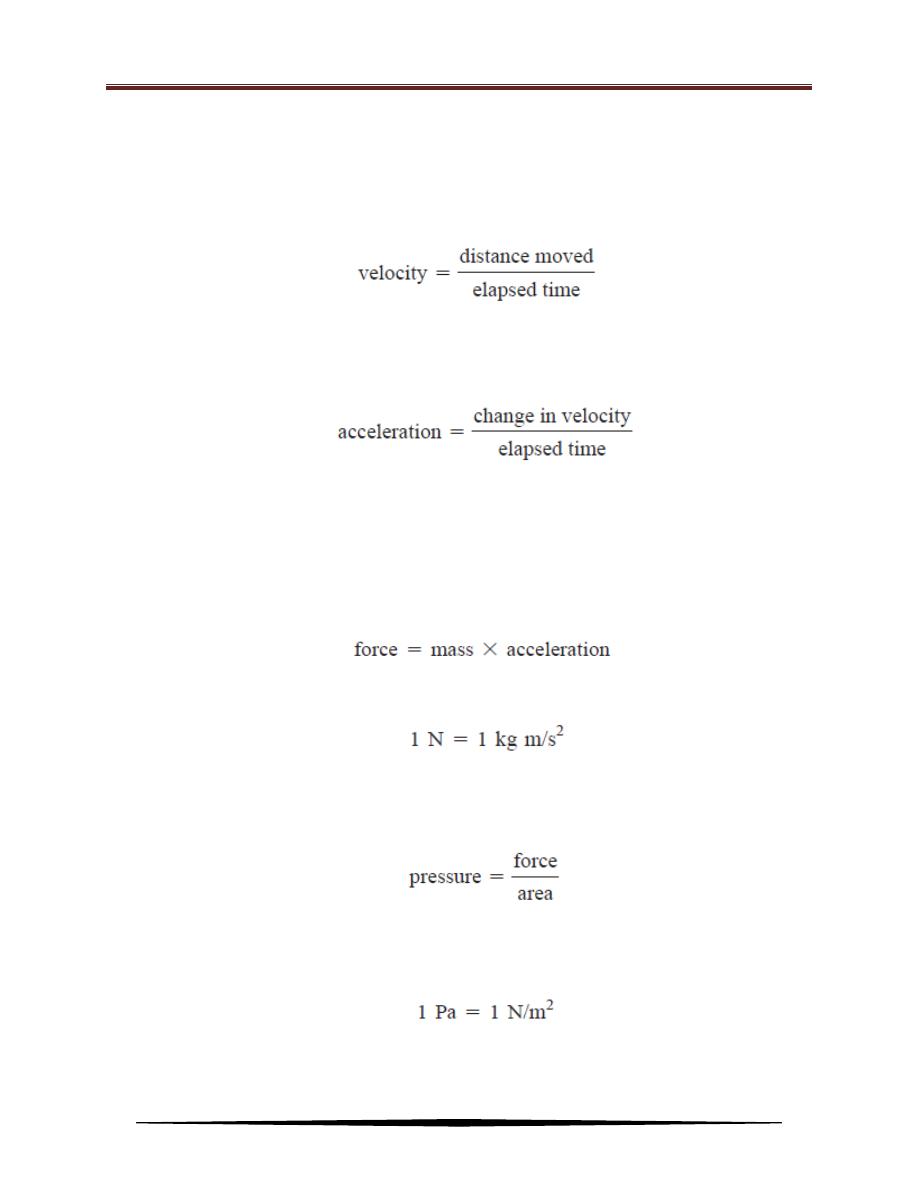
Chemistry for 1st class/Physics
Dr. Alaa Hussein Jalil
9
SI Units of Pressure
We begin with velocity and acceleration. Velocity is defined as the change in
distance with elapsed time; that is,
The SI unit for velocity is m/s, although we also use cm/s.
Acceleration is the change in velocity with time, or
Acceleration is measured in m/s
2
(or cm/s
2
).
The second law of motion, formulated by Sir Isaac Newton in the late
seventeenth century, defines another term, from which the units of pressure are
derived, namely, force. According to this law,
In this context, the SI unit of force is the newton (N), where
Finally, we define pressure as force applied per unit area ˗ pounds per
square inch (lb/in.
2
), commonly known as psi:
The SI unit of pressure is the pascal (Pa), defined as one newton per square
meter:
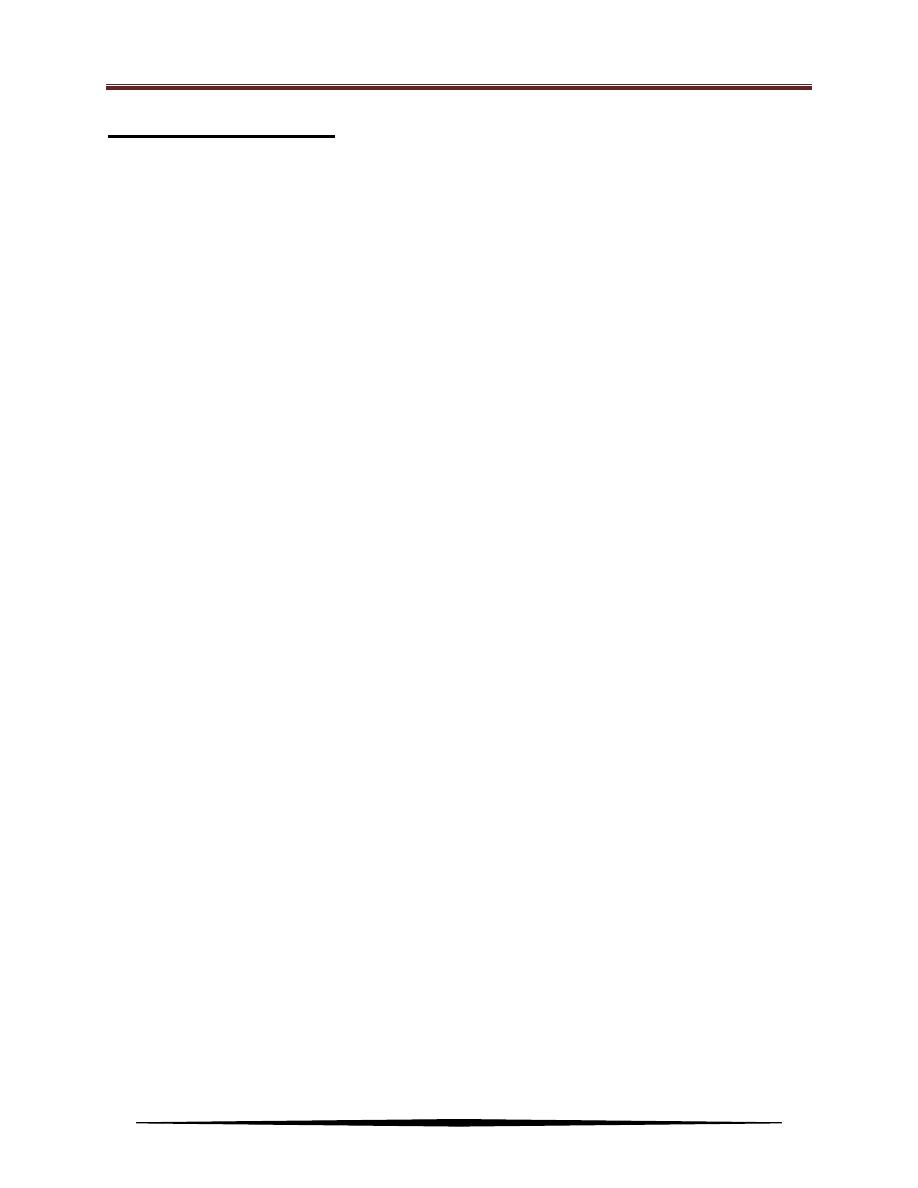
Chemistry for 1st class/Physics
Dr. Alaa Hussein Jalil
10
Atmospheric Pressure
The atoms and molecules of the gases in the atmosphere, like those of all
other matter, are subject to Earth’s gravitational pull. As a consequence, the
atmosphere is much denser near the surface of Earth than at high altitudes. In fact,
the density of air decreases very rapidly with increasing distance from Earth. Not
surprisingly, the denser the air is, the greater the pressure it exerts. The force
experienced by any area exposed to Earth’s atmosphere is equal to the weight of
the column of air above it. Atmospheric pressure is the pressure exerted by Earth’s
atmosphere. The actual value of atmospheric pressure depends on location,
temperature, and weather conditions.
Does atmospheric pressure only act downward, as you might infer from its
definition? Imagine what would happen, then, if you were to hold a piece of paper
tight with both hands above your head. You might expect the paper to bend due to
the pressure of air acting on it, but this does not happen. The reason is that air, like
water, is a fluid. The pressure exerted on an object in a fluid comes from all
directions—downward and upward, as well as from the left and from the right. At
the molecular level, air pressure results from collisions between the air molecules
and any surface with which they come in contact. The magnitude of pressure
depends on how often and how strongly the molecules impact the surface. It turns
out that there are just as many molecules hitting the paper from the top as there are
from underneath, so the paper stays flat.
How is atmospheric pressure measured? The barometer is probably the most
familiar instrument for measuring atmospheric pressure. A simple barometer
consists of a long glass tube, closed at one end and filled with mercury. If the tube
is carefully inverted in a dish of mercury so that no air enters the tube, some
mercury will flow out of the tube into the dish, creating a vacuum at the top.
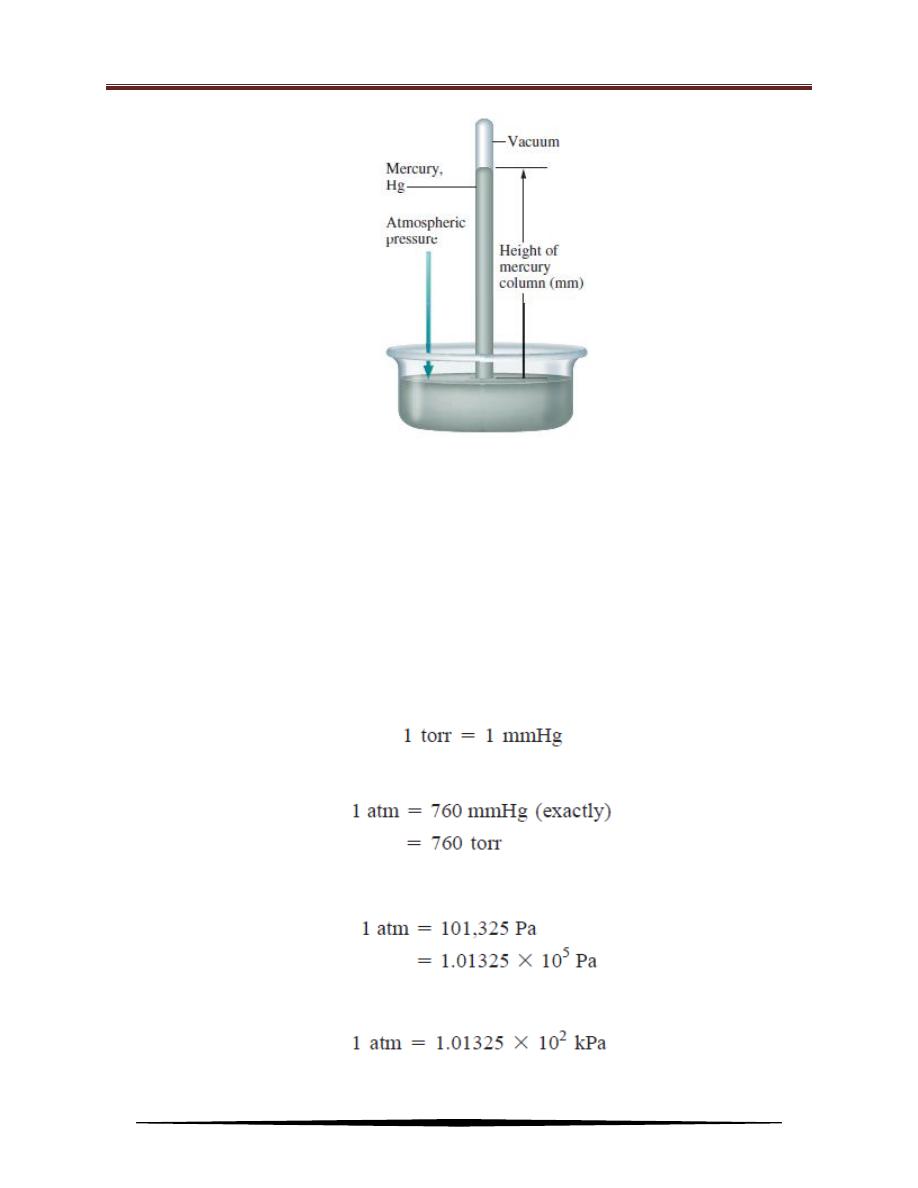
Chemistry for 1st class/Physics
Dr. Alaa Hussein Jalil
11
The weight of the mercury remaining in the tube is supported by
atmospheric pressure acting on the surface of the mercury in the dish. Standard
atmospheric pressure (1 atm) is equal to the pressure that supports a column of
mercury exactly 760 mm (or 76 cm) high at 0
o
C at sea level. In other words, the
standard atmosphere equals a pressure of 760 mmHg, where mmHg represents the
pressure exerted by a column of mercury 1 mm high. The mmHg unit is also called
the torr, after the Italian scientist Evangelista Torricelli, who invented the
barometer. Thus,
and
The relation between atmospheres and pascals is:
and because 1000 Pa = 1 kPa (kilopascal)
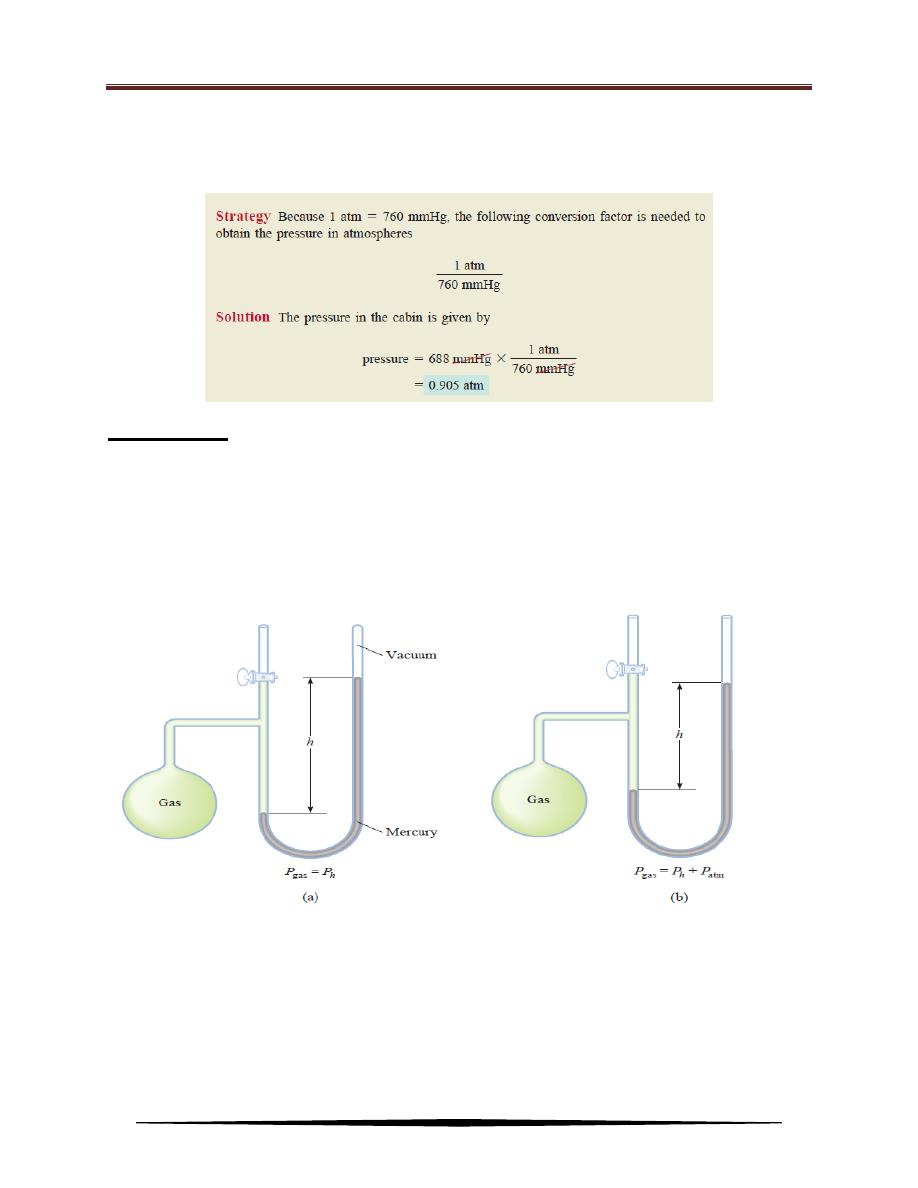
Chemistry for 1st class/Physics
Dr. Alaa Hussein Jalil
12
Example: The pressure outside a jet plane flying at high altitude falls considerably below
standard atmospheric pressure. Therefore, the air inside the cabin must be
pressurized to protect the passengers. What is the pressure in atmospheres in the
cabin if the barometer reading is 688 mmHg?
Manometer
A manometer is a device used to measure the pressure of gases other than
the atmosphere. The principle of operation of a manometer is similar to that of a
barometer. There are two types of manometers as shown in Figure below. The
closed-tube manometer is normally used to measure pressures below atmospheric
pressure [Figure (a)], whereas the open-tube manometer is better suited for
measuring pressures equal to or greater than atmospheric pressure [Figure (b)].
Nearly all barometers and most manometers use mercury as the working
fluid, despite the fact that it is a toxic substance with a harmful vapor. The reason
is that mercury has a very high density (13.6 g/mL) compared with most other
liquids. Because the height of the liquid in a column is inversely proportional to the
liquid’s density, this property enables the construction of manageably small
barometers and manometers.
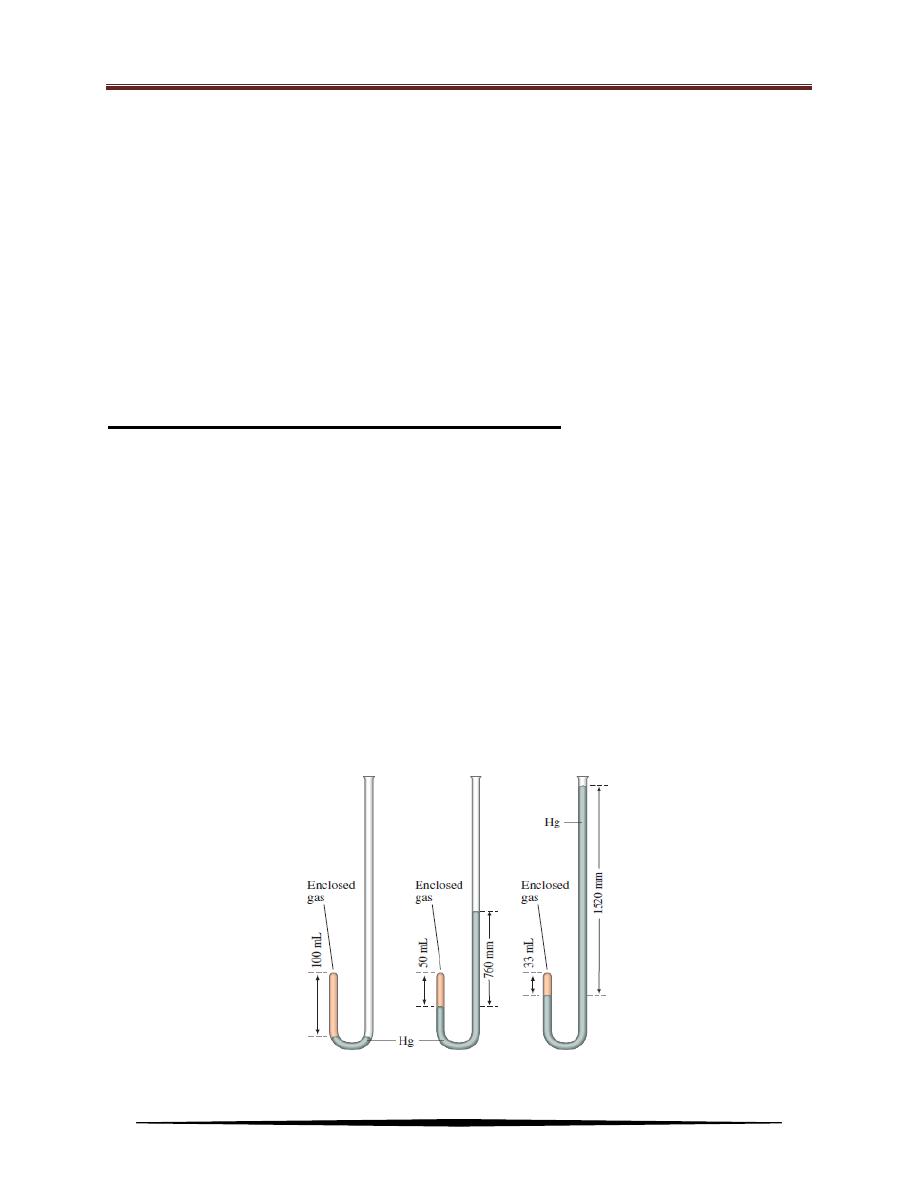
Chemistry for 1st class/Physics
Dr. Alaa Hussein Jalil
13
Empirical Gas Laws
All gases under moderate conditions behave quite simply with respect to
pressure, temperature, volume, and molar amount. By holding any two of these
physical properties constant, it is possible to show a simple relationship between
the other two. Throughout history there have been multiple versions of gas laws
developed and named after many different people. Boyle’s Law (1662), Charles’s
Law (1802), and Avogadro’s Law (1811) are a few examples.
Boyle’s Law: Relating Volume and Pressure
One characteristic property of a gas is its compressibility—its ability to be
squeezed into a smaller volume by the application of pressure. By comparison,
liquids and solids are relatively incompressible. The compressibility of gases was
first studied quantitatively by Robert Boyle in 1661. When he poured mercury into
the open end of a J-shaped tube, the volume of the enclosed gas decreased (see
Figure below). Each addition of mercury increased the pressure on the gas,
decreasing its volume. From such experiments, he formulated the law now known
by his name. According to Boyle’s law, the volume of a sample of gas at a given
temperature varies inversely with the applied pressure.
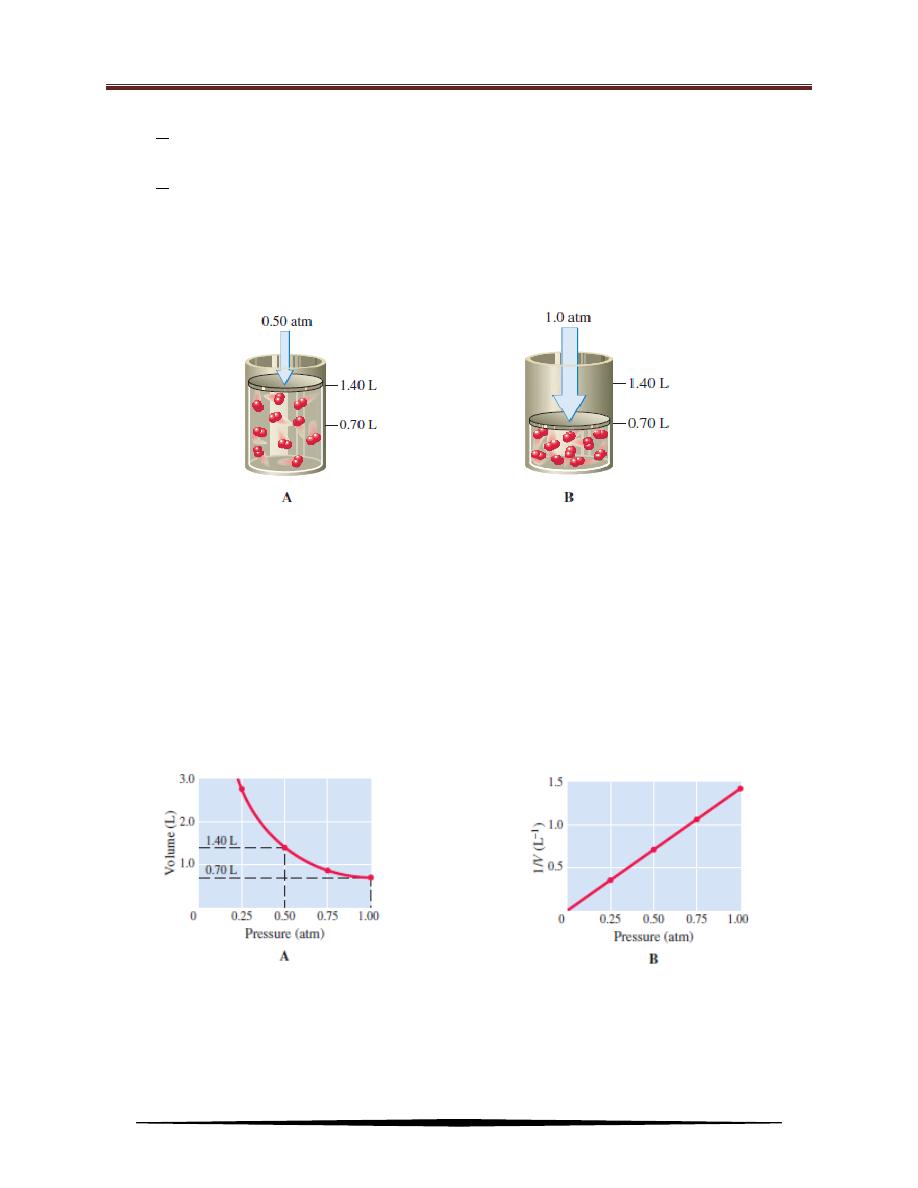
Chemistry for 1st class/Physics
Dr. Alaa Hussein Jalil
14
P
1
, meaning "Volume is directly proportional to 1 divided by Pressure", or
V
1
, meaning "Pressure is directly proportional to 1 divided by Volume"
Another way to describing it is saying that their products are constant.
PV = k
When pressure goes up, volume goes down. When volume goes up, pressure
goes down.
Model of gas pressure–volume relationship at a constant temperature:
(A) When a 1.000-g sample of O
2
gas at 0
o
C is placed in a container at a pressure
of 0.50 atm, it occupies a volume of 1.40 L.
(B) When the pressure on the O
2
sample is doubled to 1.0 atm, the volume is
reduced to 0.70 L, which is half the original volume.
By plotting the volume of the oxygen at different pressures, you obtain a graph
showing the inverse relationship of P and V.
Gas pressure–volume relationship:
(A) Plot of volume vs. pressure for a sample
of oxygen. The volume (of 1.000 g O
2
at
0
o
C)
decreases
with
increasing
pressure. When the pressure is doubled
(from 0.50 atm to 1.00 atm), the volume
is halved (from 1.40 L to 0.70 L).
(B) Plot of 1/V vs. pressure (at constant
temperature) for the same sample.
The straight line indicates that volume
varies inversely with pressure.
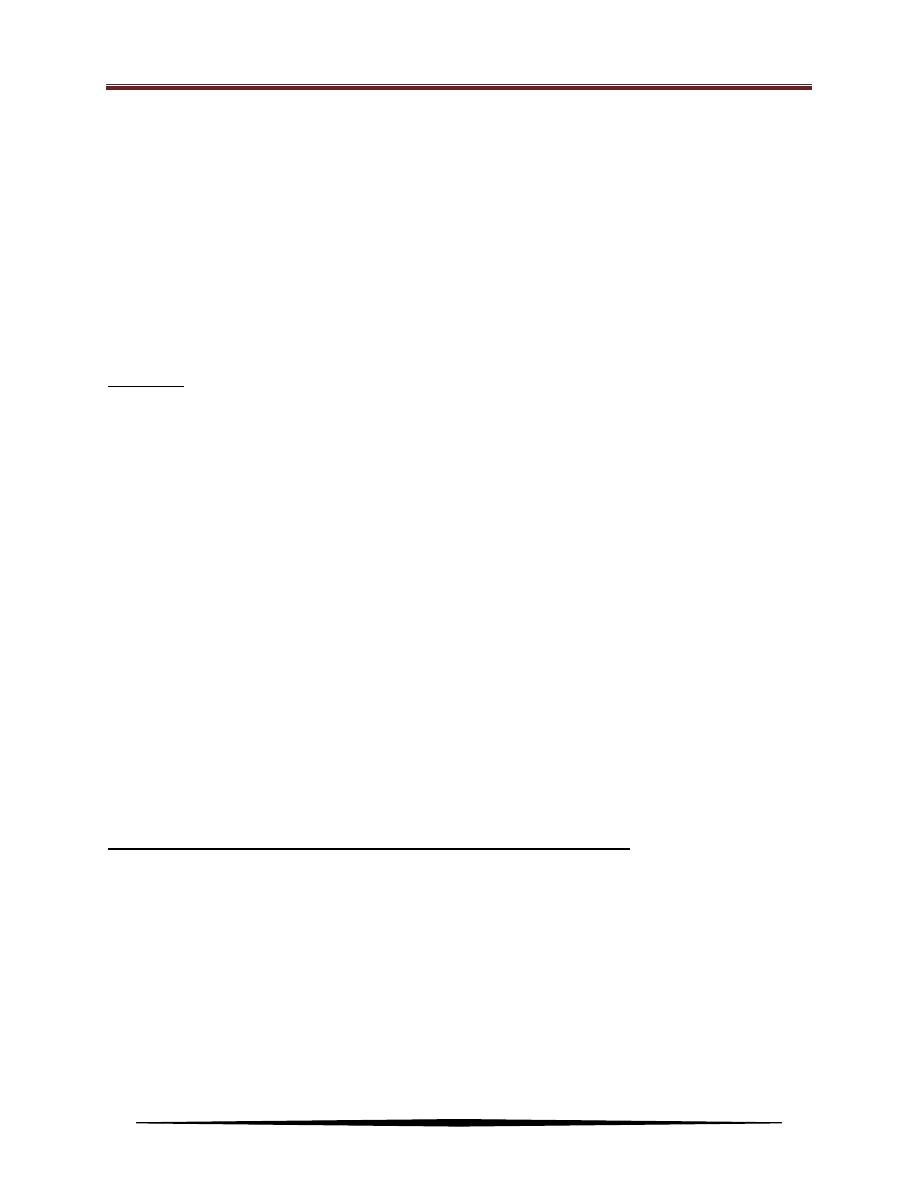
Chemistry for 1st class/Physics
Dr. Alaa Hussein Jalil
15
From the equation above, this can be derived:
P
1
V
1
= P
2
V
2
= P
3
V
3
etc.
This equation states that the product of the initial volume and pressure is
equal to the product of the volume and pressure after a change in one of them
under constant temperature. For example, if the initial volume was 500 mL at a
pressure of 760 torr, when the volume is compressed to 450 mL, what is the
pressure?
Solution:
P
1
V
1
= P
2
V
2
(760 torr)(500 mL) = P
2
(450 mL)
760 torr x 500 mL/450 mL = P
2
844 torr
P
2
= 844 torr.
A volume of air occupying 12.0 dm3 at 98.9 kPa is compressed to a pressure of
119.0 kPa. The temperature remains constant. What is the new volume?
A volume of carbon dioxide gas, CO
2
, equal to 20.0 L was collected at 23
o
C
and 1.00 atm pressure. What would be the volume of carbon dioxide collected
at 23
o
C and 0.830 atm?
In fact, all gases follow Boyle’s law at low to moderate pressures but deviate
from this law at high pressures. The extent of deviation depends on the gas.
Charles’s Law: Relating Volume and Temperature
Boyle’s law depends on the temperature of the system remaining constant.
But suppose the temperature changes: How does a change in temperature affect the
volume and pressure of a gas? Let’s first look at the effect of temperature on the
volume of a gas. The earliest investigators of this relationship were French
scientists, Jacques Charles. His study showed that, at constant pressure, the volume
of a gas sample expands when heated and contracts when cooled:
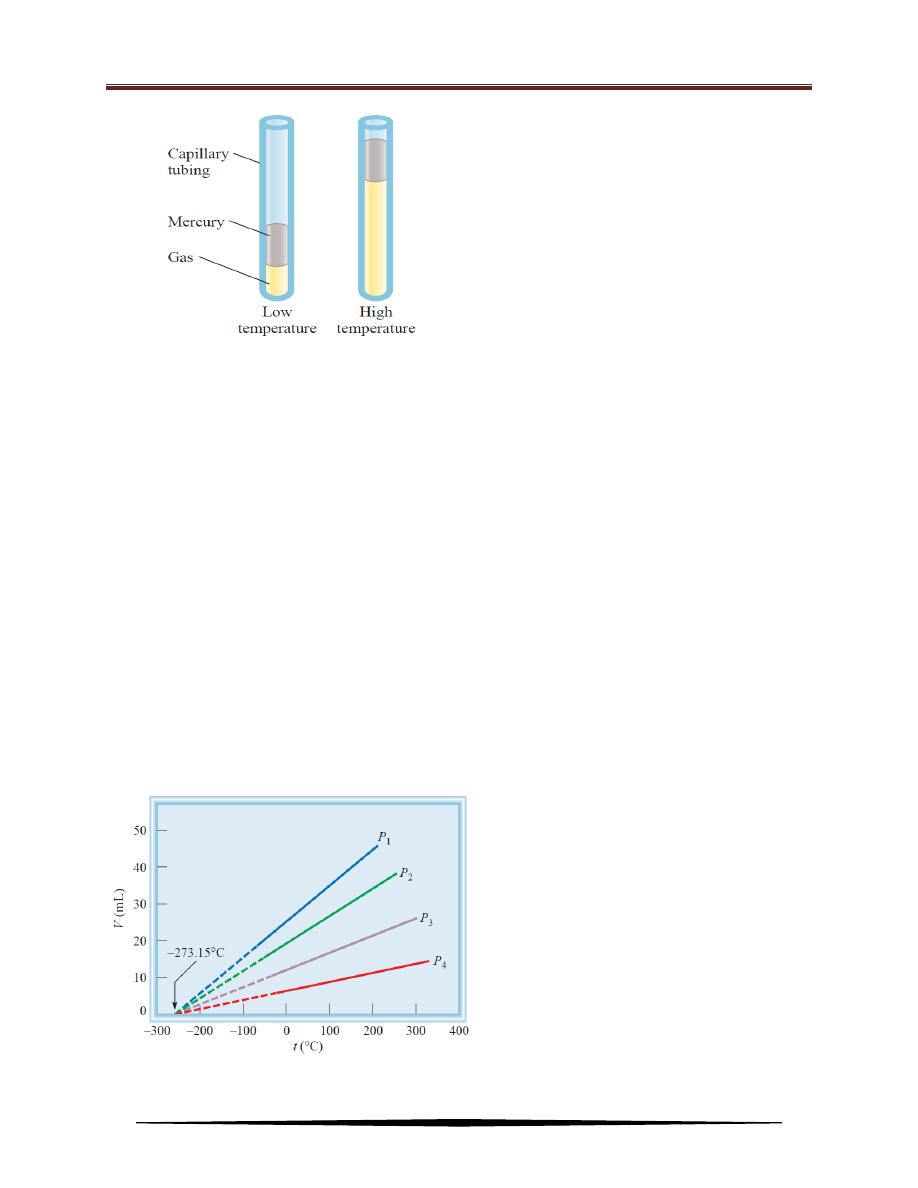
Chemistry for 1st class/Physics
Dr. Alaa Hussein Jalil
16
The quantitative relations involved in changes in gas temperature and
volume turn out to be remarkably consistent. For example, we observe an
interesting phenomenon when we study the temperature-volume relationship at
various pressures. At any given pressure, the plot of volume versus temperature
yields a straight line. By extending the line to zero volume, we find the intercept on
the temperature axis to be ‒273.15
o
C. At any other pressure, we obtain a different
straight line for the volume-temperature plot, but we get the same zero-volume
temperature intercept at ‒273.15
o
C (see Figure below). This seems to say that if
the substances remain gaseous, the volumes occupied will be zero at ‒273.15
o
C.
This could not happen, however; all gases liquefy before they reach this
temperature, and Charles’s law does not apply to liquids. These extrapolations do
show that we can express the volume variation of a gas with temperature more
simply by choosing a different thermometer scale.
Variation of the volume of a gas sample
with temperature, at constant pressure.
The pressure exerted on the gas is the sum
of the atmospheric pressure and the
pressure due to the weight of the mercury.
Variation of the volume of a gas sample
with temperature, at constant pressure.
Each line represents the variation at a
certain pressure. The pressures increase
from P
1
to P
4
. All gases ultimately
condense (become liquids) if they are
cooled to sufficiently low temperatures;
the solid portions of the lines represent the
temperature
region
above
the
condensation point. When these lines are
extrapolated, or extended (the dashed
portions), they all intersect at the point
representing
zero
volume
and
a
temperature of ‒273.15
o
C.
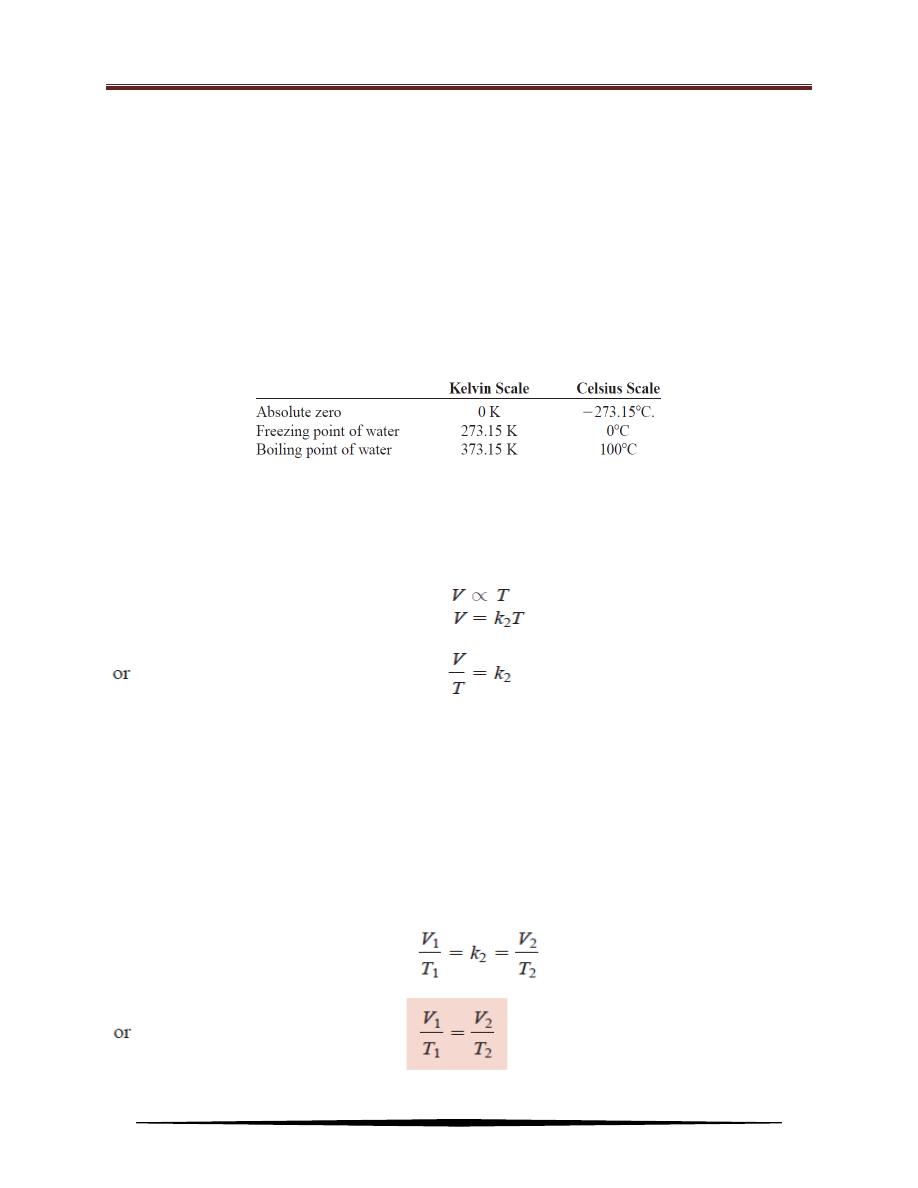
Chemistry for 1st class/Physics
Dr. Alaa Hussein Jalil
17
In 1848 the Scottish physicist Lord Kelvin realized the significance of this
phenomenon. He identified ‒273.15
o
C as absolute zero, theoretically the lowest
attainable temperature. Then he set up an absolute temperature scale, now called
the Kelvin temperature scale, with absolute zero as the starting point. On the
Kelvin scale, one kelvin (K) is equal in magnitude to one degree Celsius. The only
difference between the absolute temperature scale and the Celsius scale is that the
zero position is shifted. Important points on the two scales match up as follows:
In most calculations we will use 273 instead of 273.15 as the term relating K
and
o
C.
The dependence of the volume of a gas on temperature is given by:
where k
2
is the proportionality constant. Equation above is known as
Charles’s law, which states that the volume of a fixed amount of gas maintained at
constant pressure is directly proportional to the absolute temperature of the gas.
For pressure-volume relationships at constant temperature, we can compare two
sets of volume-temperature conditions for a given sample of gas at constant
pressure.
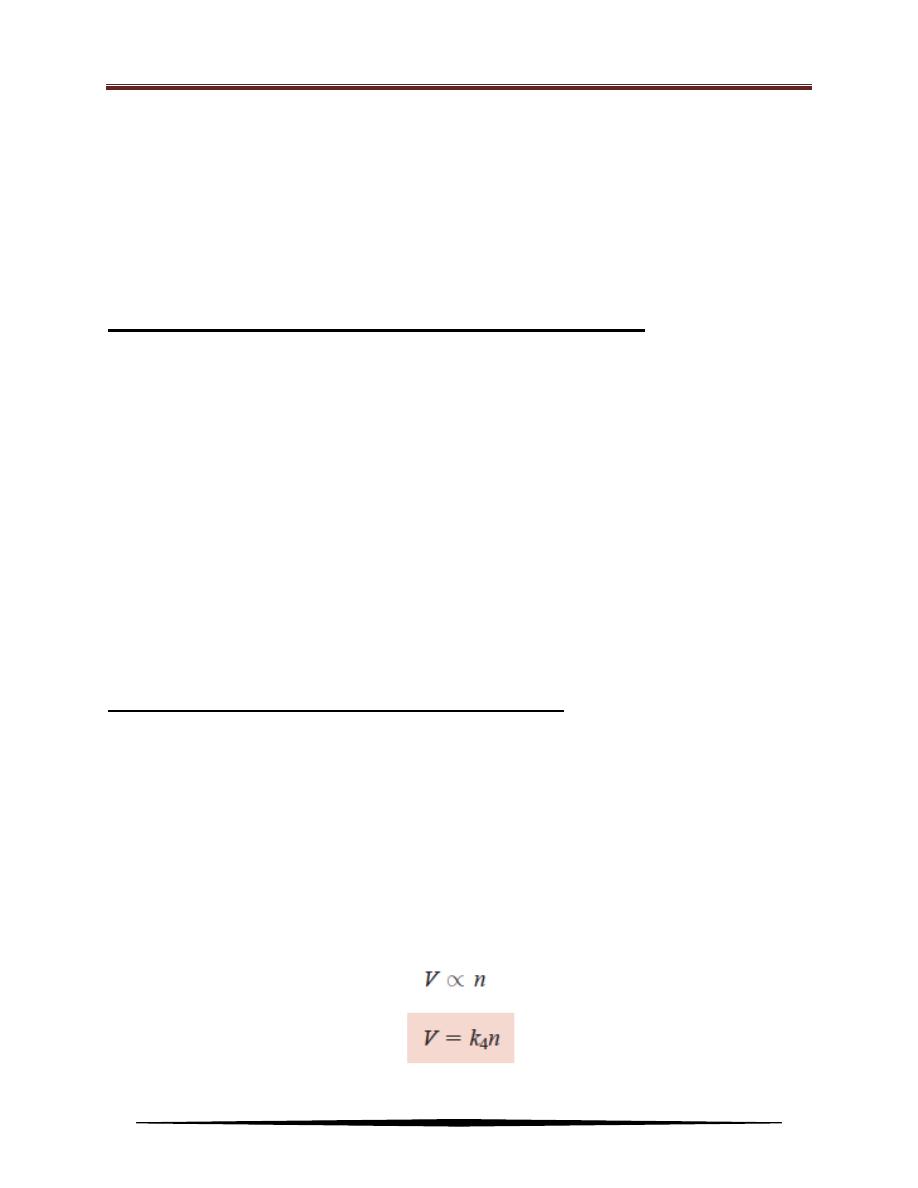
Chemistry for 1st class/Physics
Dr. Alaa Hussein Jalil
18
where V
1
and V
2
are the volumes of the gas at temperatures T
1
and T
2
(both in
kelvins), respectively. As the volume goes up, the temperature also goes up, and
vice-versa. Also same as before, initial and final volumes and temperatures under
constant pressure can be calculated.
V
1
/ T
1
= V
2
/ T
2
= V
3
/ T
3
etc.
Gay-Lussac's Law: The Pressure Temperature Law
This law states that the pressure of a given amount of gas held at constant
volume is directly proportional to the Kelvin temperature.
Same as before, a constant can be put in:
P / T = k
As the pressure goes up, the temperature also goes up, and vice-versa. Also same
as before, initial and final volumes and temperatures under constant pressure can
be calculated.
P
1
/ T
1
= P
2
/ T
2
= P
3
/ T
3
etc.
Avogadro's Law: The Volume Amount Law
The work of the Italian scientist Amedeo Avogadro complemented the
studies of Boyle, Charles, and Gay-Lussac. In 1811 he published a hypothesis
stating that at the same temperature and pressure, equal volumes of different gases
contain the same number of molecules (or atoms if the gas is monatomic). It
follows that the volume of any given gas must be proportional to the number of
moles of molecules present; that is:
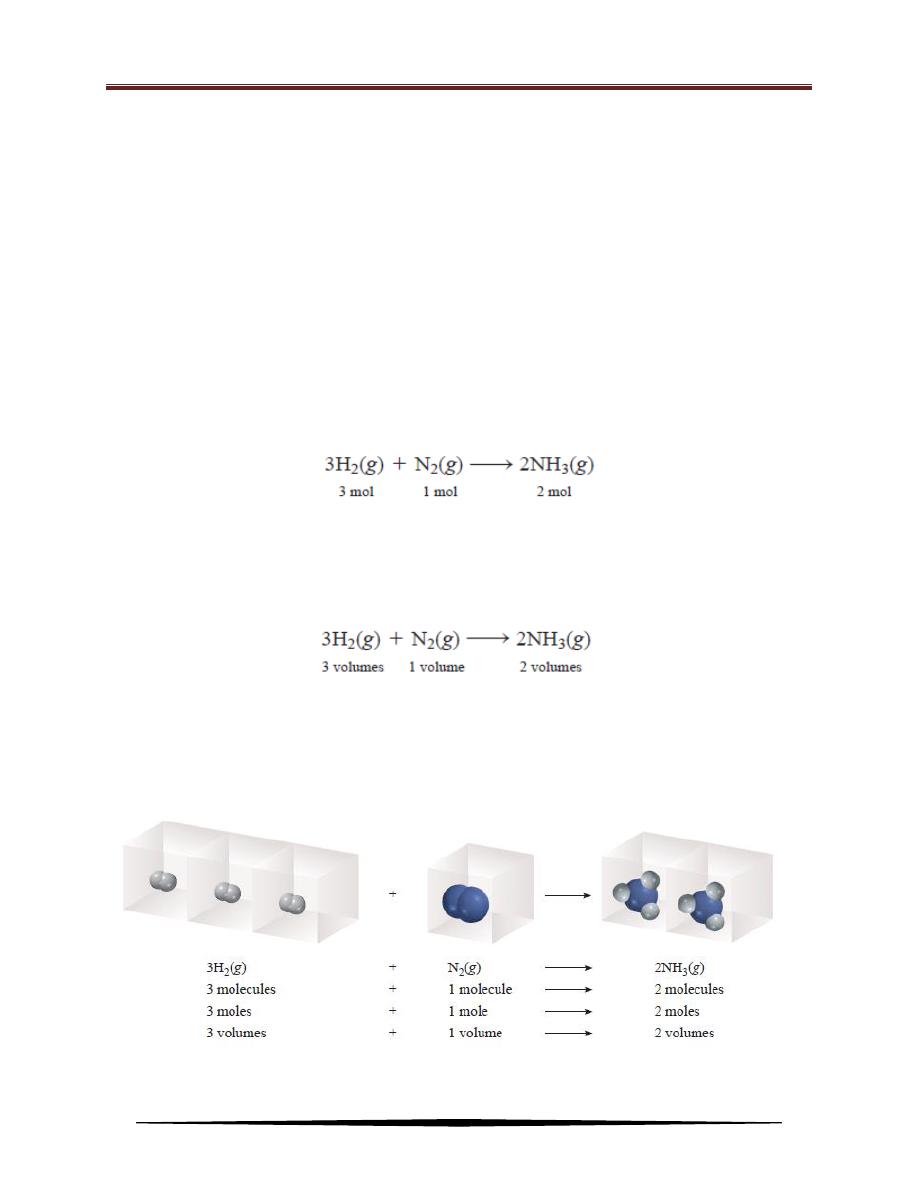
Chemistry for 1st class/Physics
Dr. Alaa Hussein Jalil
19
where n represents the number of moles and k
4
is the proportionality constant. This
equation is the mathematical expression of Avogadro’s law, which states that at
constant pressure and temperature, the volume of a gas is directly proportional to
thenumber of moles of the gas present.
According to Avogadro’s law we see that when two gases react with each
other, their reacting volumes have a simple ratio to each other. If the product is a
gas, its volume is related to the volume of the reactants by a simple ratio. For
example, consider the synthesis of ammonia from molecular hydrogen and
molecular nitrogen:
Because, at the same temperature and pressure, the volumes of gases are
directly proportional to the number of moles of the gases present, we can now
write:
The volume ratio of molecular hydrogen to molecular nitrogen is 3:1, and
that of ammonia (the product) to molecular hydrogen and molecular nitrogen
combined (the reactants) is 2:4, or 1:2 (see Figure below).
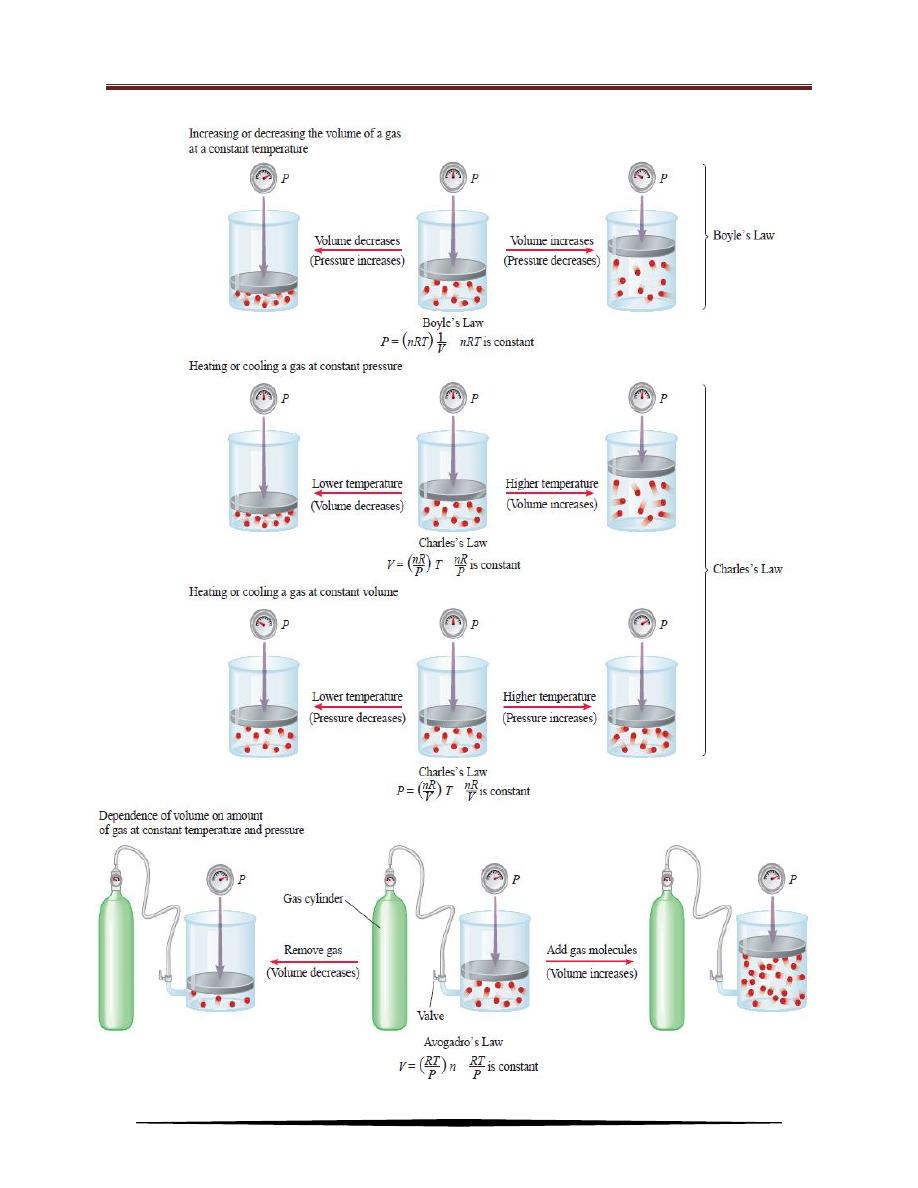
Chemistry for 1st class/Physics
Dr. Alaa Hussein Jalil
20
Schematic illustrations of Boyle’s law, Charles’s law, and Avogadro’s law.
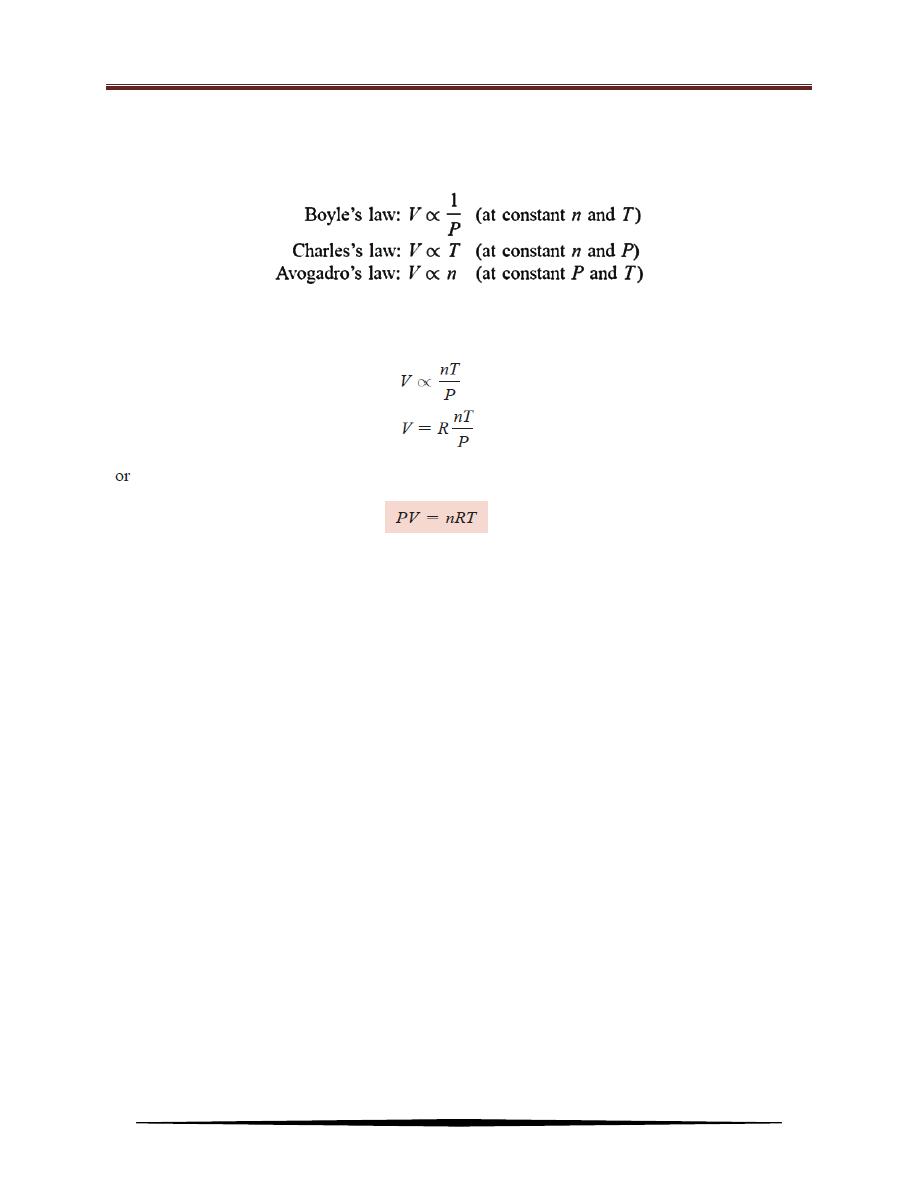
Chemistry for 1st class/Physics
Dr. Alaa Hussein Jalil
21
The Ideal Gas Law
The gas laws that have been discussed so far, can be summarized:
All three expressions can combine to form a single master equation for the
behavior of gases:
where R, the proportionality constant, is called the gas constant. This equation,
which is called the ideal gas equation, describes the relationship among the four
variables P, V, T, and n. An ideal gas is a hypothetical gas whose pressure-
volume-temperature behavior can be completely accounted for by the ideal gas
equation. The molecules of an ideal gas do not attract or repel one another, and
their volume is negligible compared with the volume of the container. Although
there is no such thing in nature as an ideal gas, discrepancies in the behavior of real
gases over reasonable temperature and pressure ranges do not significantly affect
calculations. Thus, the ideal gas equation can safely use to solve many gas
problems.
Before the ideal gas equation can apply to a real system, the gas constant R
must evaluated. At 0
o
C (273.15 K) and 1 atm pressure, many real gases behave
like an ideal gas. Experiments show that under these conditions, 1 mole of an ideal
gas occupies 22.414 L. The conditions 0
o
C and 1 atm are called standard
temperature and pressure, often abbreviated STP. From the ideal gas equation, we
can write:
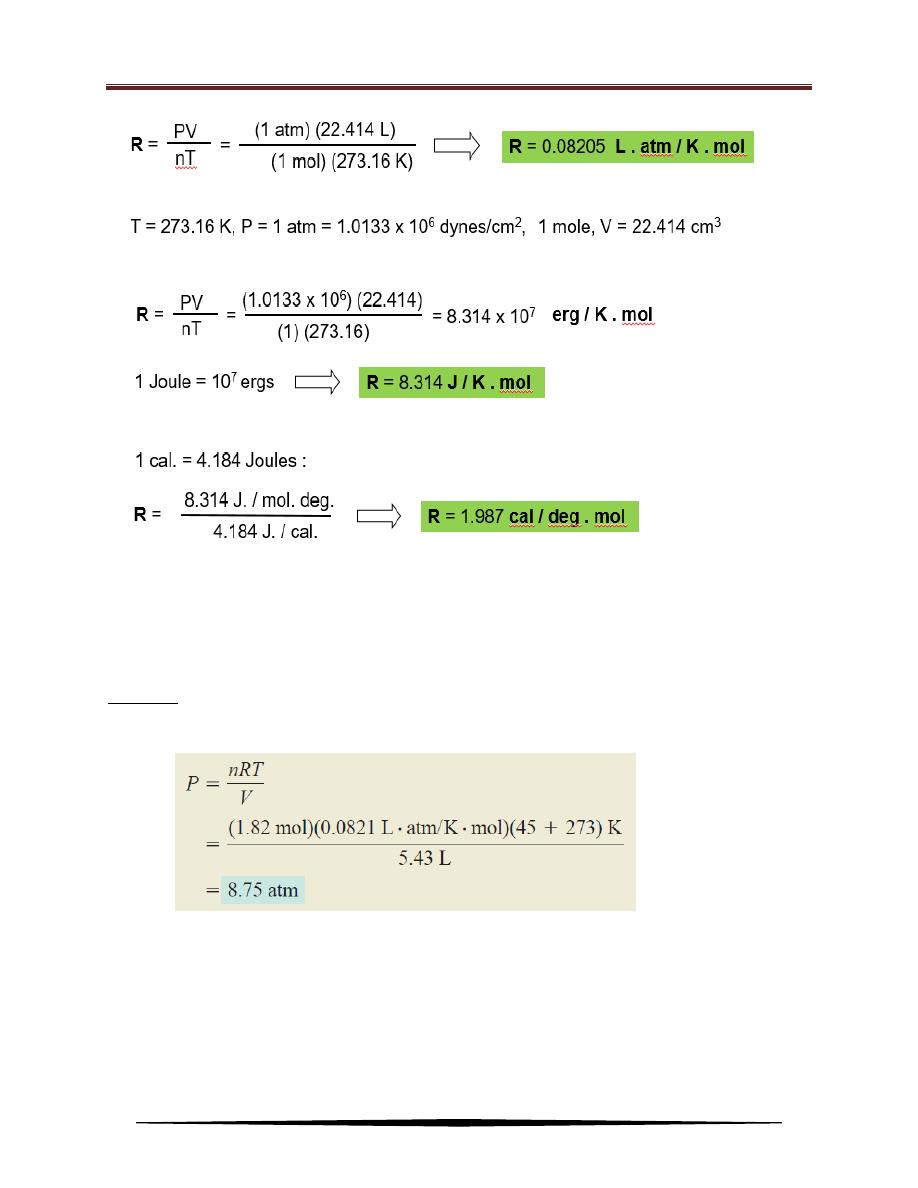
Chemistry for 1st class/Physics
Dr. Alaa Hussein Jalil
22
Example: Sulfur hexafluoride (SF
6
) is a colorless, odorless, very unreactive gas.
Calculate the pressure (in atm) exerted by 1.82 moles of the gas in a steel
vessel of volume 5.43 L at 45
o
C.
Solution: Because no changes in gas properties occur, the ideal gas equation can
use to calculate the pressure. Rearranging:
The ideal gas equation is useful for problems that do not involve changes in P, V,
T, and n for a gas sample. When conditions change, a modified form of the ideal
gas equation that takes into account the initial and final conditions must employ.
The modified equation derives as follows:
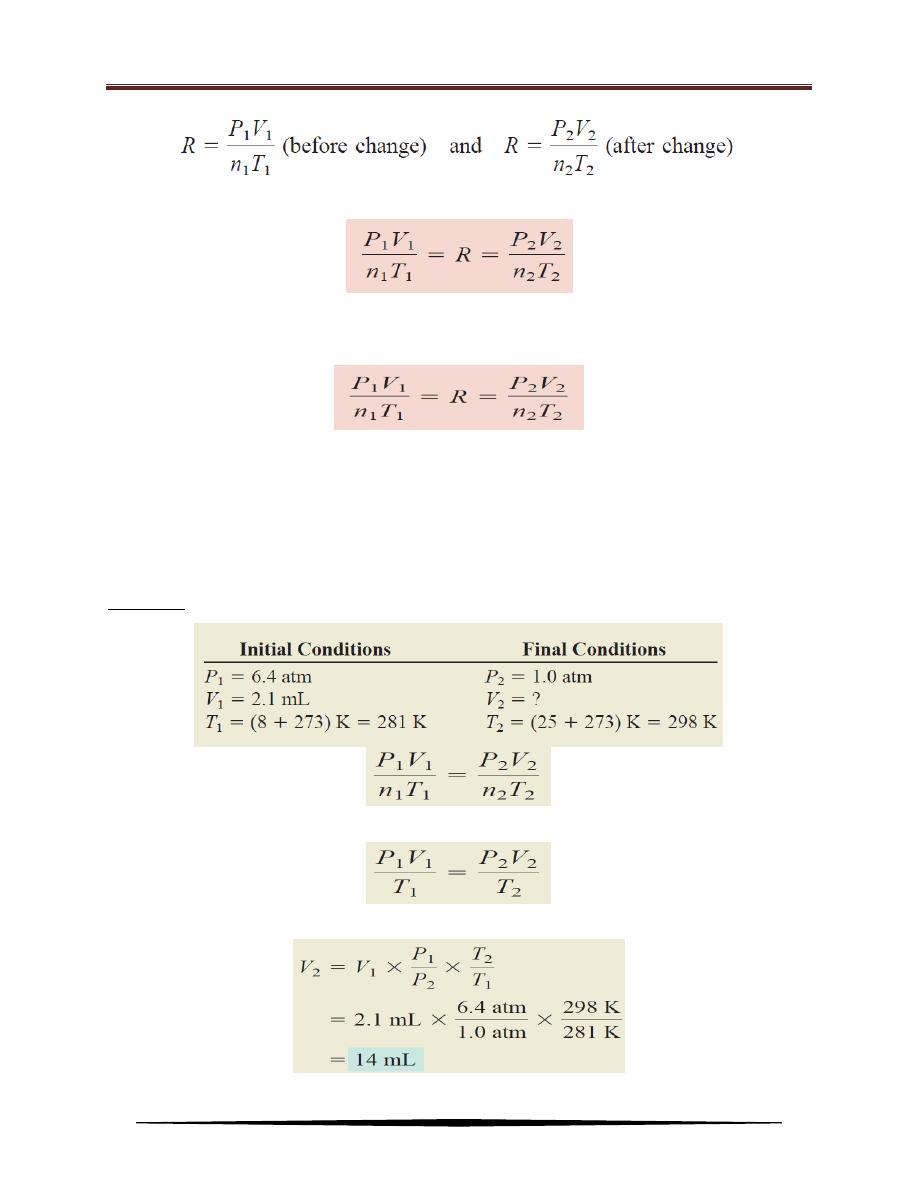
Chemistry for 1st class/Physics
Dr. Alaa Hussein Jalil
23
Therefore,
If n
1
= n
2
, as is usually the case because the amount of gas normally does not
change, the equation then becomes:
Example: A small bubble rises from the bottom of a lake, where the temperature
and pressure are 8
o
C and 6.4 atm, to the water’s surface, where the
temperature is 25
o
C and the pressure is 1.0 atm. Calculate the final
volume (in mL) of the bubble if its initial volume was 2.1 mL.
Solution: The given information is summarized:
The amount of air in the bubble remains constant, that is, n
1
= n
2
so that:
Rearranging equation above gives:
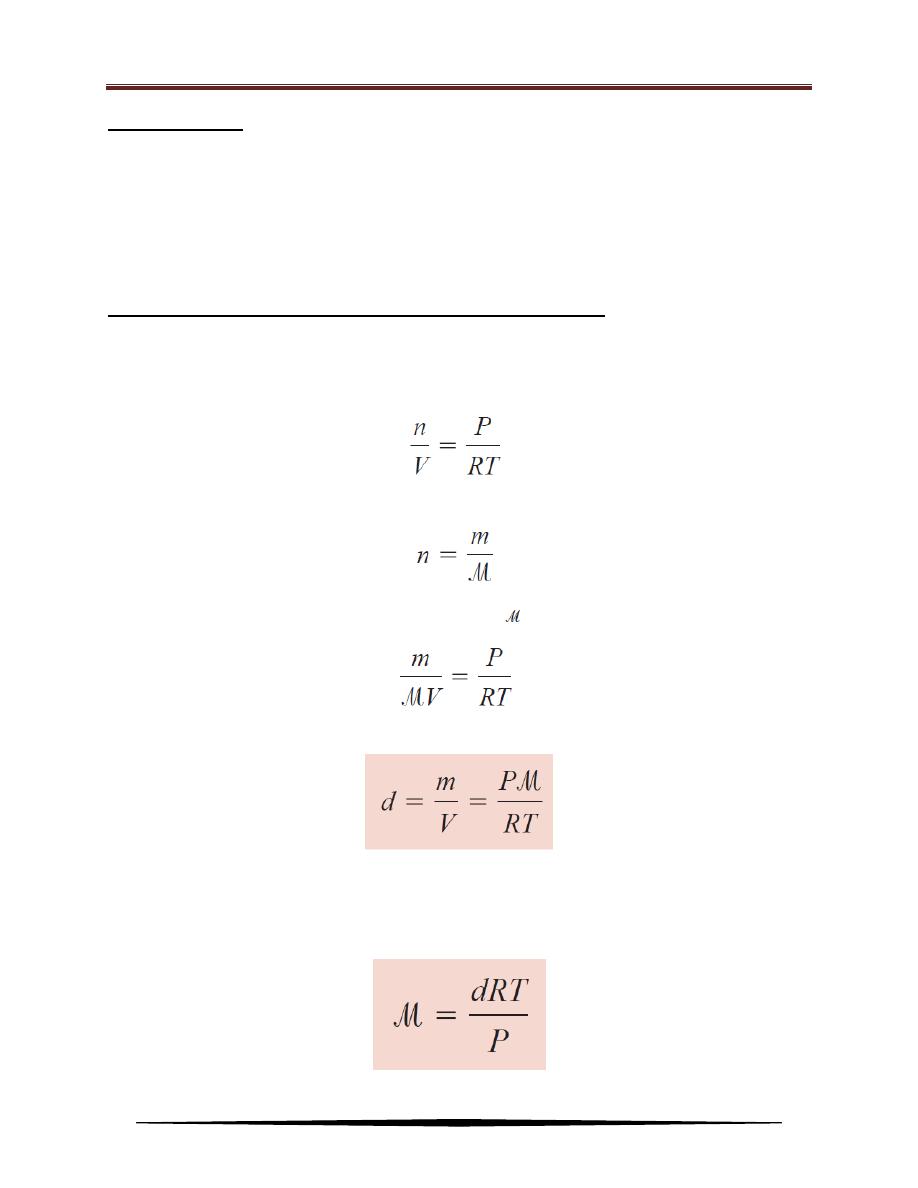
Chemistry for 1st class/Physics
Dr. Alaa Hussein Jalil
24
Practice Exercise:
What is the volume (in liters) occupied by 49.8 g of HCl at STP?
A gas initially at 4.0 L, 1.2 atm, and 66
o
C undergoes a change so that its
final volume and temperature are 1.7 L and 42
o
C. What is its final pressure?
Assume the number of moles remains unchanged.
Density and Molar Mass of a Gaseous Substance
The ideal gas equation can be applied to determine the density or molar mass
of a gaseous substance. Rearranging this equation gives:
The number of moles of the gas, n, is given by:
in which m is the mass of the gas in grams and is its molar mass. Therefore,
Because density, d, is mass per unit volume, therefore:
The equation above enables to calculate the density of a gas (given in units
of grams per liter). More often, the density of a gas can be measured, so this
equation can be rearranged to calculate the molar mass of a gaseous substance:
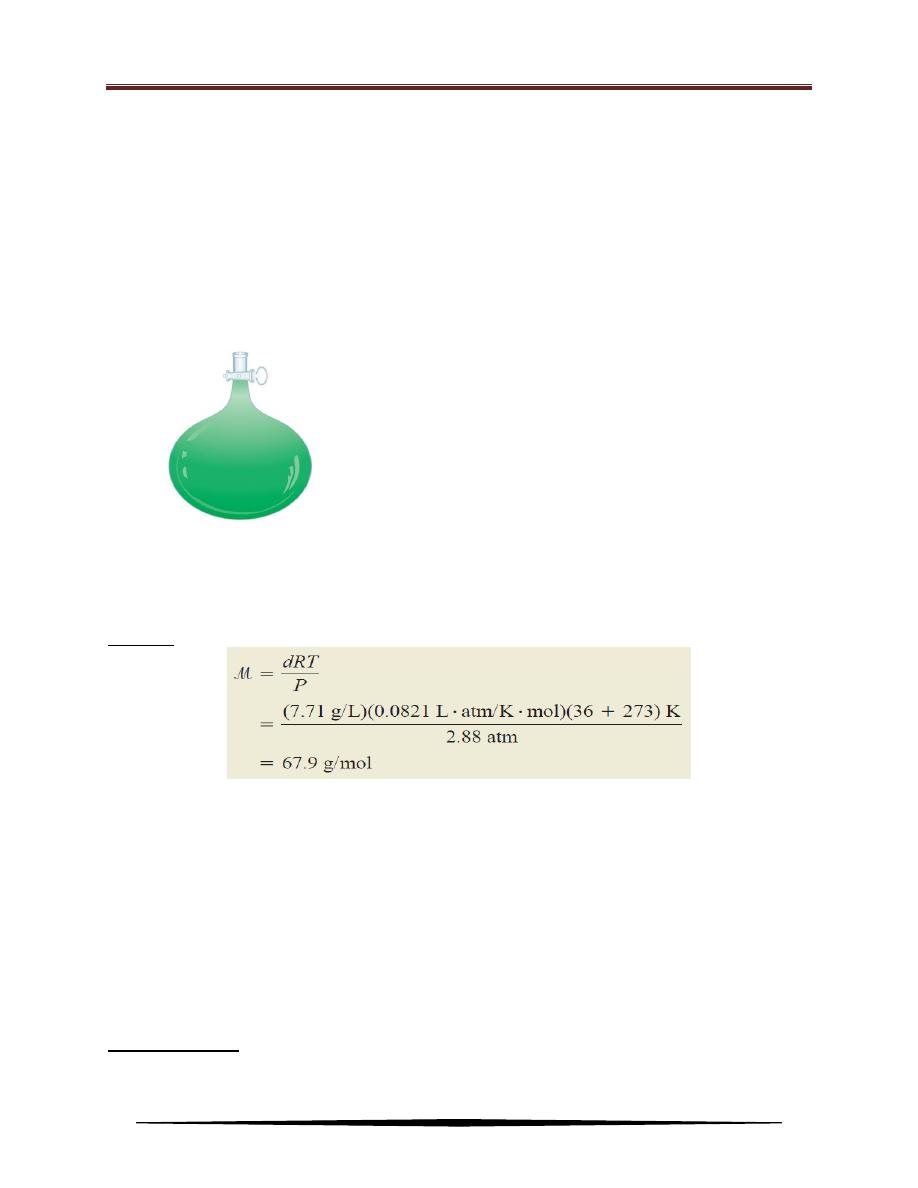
Chemistry for 1st class/Physics
Dr. Alaa Hussein Jalil
25
In a typical experiment, a bulb of known volume is filled with the gaseous
substance under study. The temperature and pressure of the gas sample are
recorded, and the total mass of the bulb plus gas sample is determined (see Figure
below). The bulb is then evacuated (emptied) and weighed again. The difference in
mass is the mass of the gas. The density of the gas is equal to its mass divided by
the volume of the bulb. Then the molar mass of the substance can be calculated
using the last equation.
Example: A chemist has synthesized a greenish-yellow gaseous compound of chlorine and
oxygen and finds that its density is 7.71 g/L at 36
o
C and 2.88 atm. Calculate the
molar mass of the compound and determine its molecular formula.
Solution:
The molecular formula of the compound can be determined by trial and
error, using only the knowledge of the molar masses of chlorine (35.45 g) and
oxygen (16.00 g). A compound containing one Cl atom and one O atom would
have a molar mass of 51.45 g, which is too low, while the molar mass of a
compound made up of two Cl atoms and one O atom is 86.90 g, which is too high.
Thus, the compound must contain one Cl atom and two O atoms and have the
formula ClO
2
, which has a molar mass of 67.45 g.
Practice Exercise: The density of a gaseous organic compound is 3.38 g/L at 40
o
C and 1.97
atm. What is its molar mass?
An apparatus for measuring the density of a gas. A
bulb of known volume is filled with the gas under study
at a certain temperature and pressure. First the bulb is
weighed, and then it is emptied (evacuated) and
weighed again. The difference in masses gives the mass
of the gas. Knowing the volume of the bulb, we can
calculate the density of the gas.
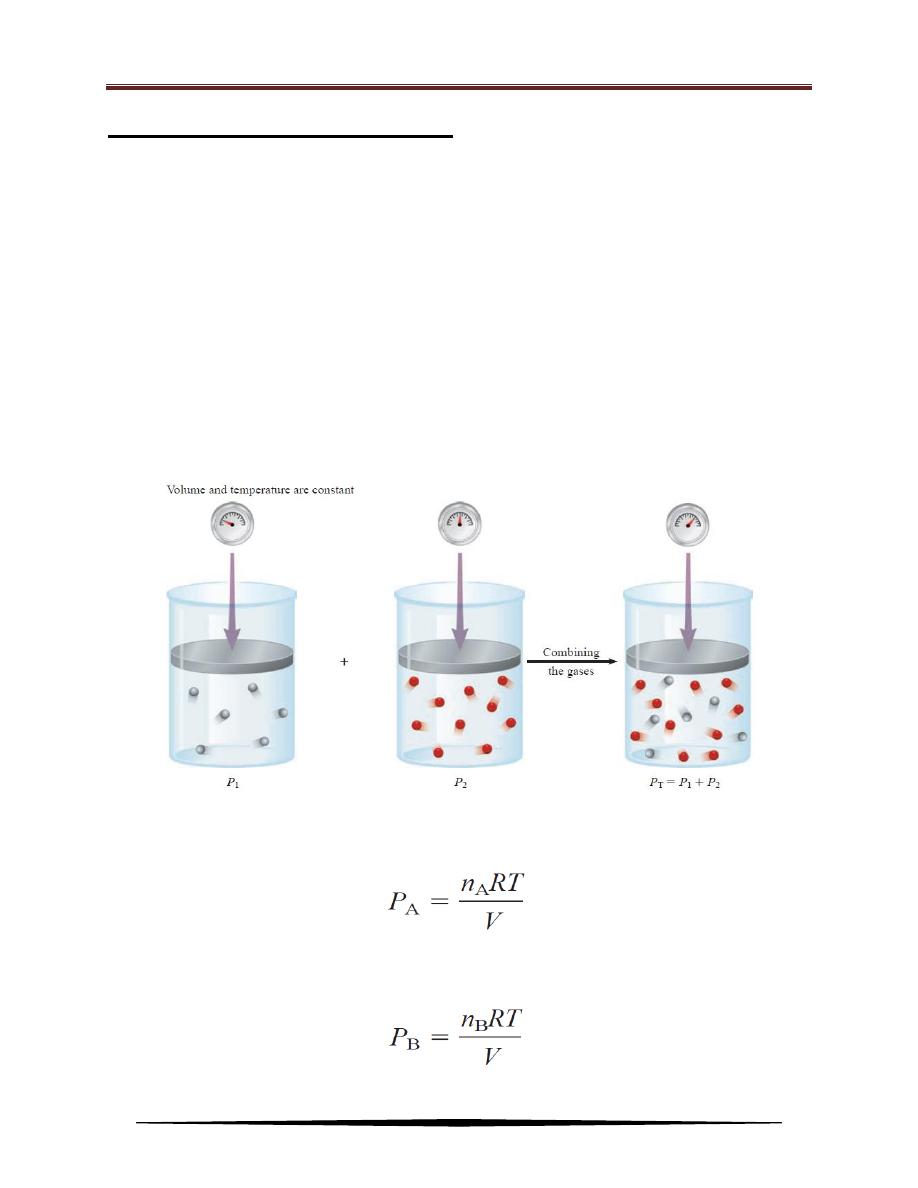
Chemistry for 1st class/Physics
Dr. Alaa Hussein Jalil
26
Dalton’s Law of Partial Pressures
The experimental studies very often involve mixtures of gases. For example,
for a study of air pollution, the pressure-volume-temperature relationship of a
sample of air, which contains several gases, may be interested. In this case, and all
cases involving mixtures of gases, the total gas pressure is related to partial
pressures, that is, the pressures of individual gas components in the mixture. In
1801 Dalton formulated a law, now known as Dalton’s law of partial pressures,
which states that the total pressure of a mixture of gases is just the sum of the
pressures that each gas would exert if it were present alone. Figure below
illustrates Dalton’s law.
Consider a case in which two gases, A and B, are in a container of volume V.
The pressure exerted by gas A, according to the ideal gas equation, is:
where n
A
is the number of moles of A present. Similarly, the pressure exerted by
gas B is:
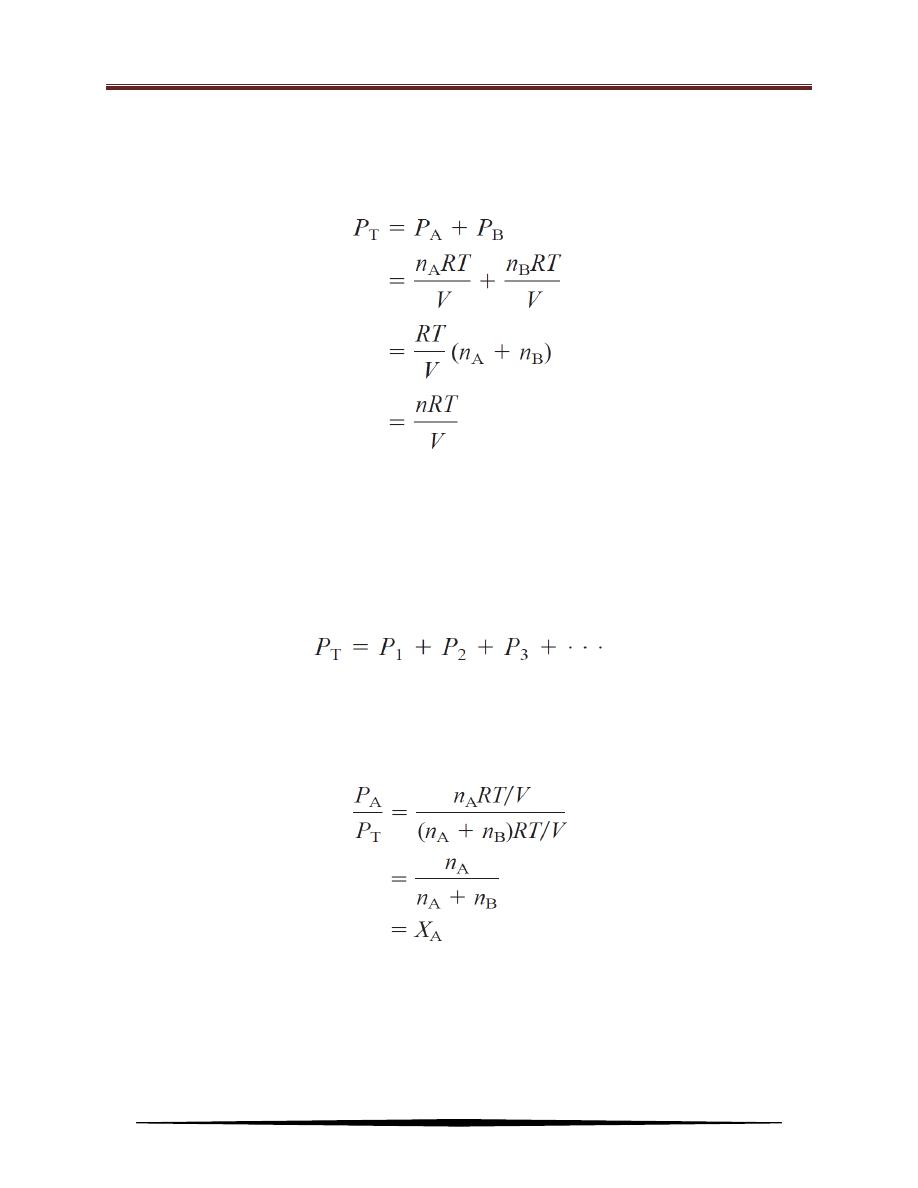
Chemistry for 1st class/Physics
Dr. Alaa Hussein Jalil
27
In a mixture of gases A and B, the total pressure P
T
is the result of the
collisions of both types of molecules, A and B, with the walls of the container.
Thus, according to Dalton’s law:
where n, the total number of moles of gases present, is given by n = n
A
+ n
B
, and
P
A
and P
B
are the partial pressures of gases A and B, respectively. For a mixture of
gases, then, P
T
depends only on the total number of moles of gas present, not on
the nature of the gas molecules.
In general, the total pressure of a mixture of gases is given by:
where P
1
, P
2
, P
3
, . . . are the partial pressures of components 1, 2, 3, . . . . To see
how each partial pressure is related to the total pressure, consider again the case of
a mixture of two gases A and B. Dividing P
A
by P
T
, can obtain:
where X
A
is called the mole fraction of A. The mole fraction is a dimensionless
quantity that expresses the ratio of the number of moles of one component to the
number of moles of all components present. In general, the mole fraction of
component i in a mixture is given by:
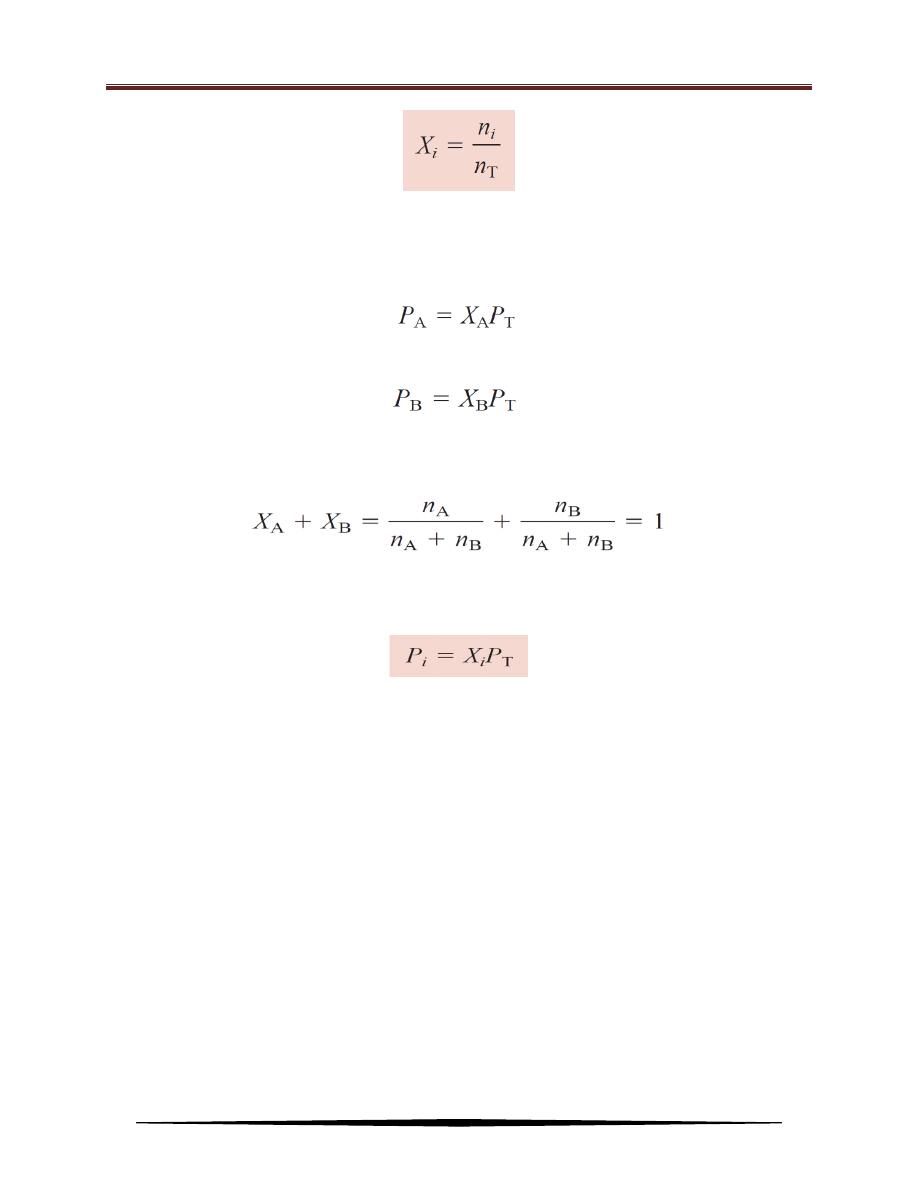
Chemistry for 1st class/Physics
Dr. Alaa Hussein Jalil
28
where n
i
and n
T
are the number of moles of component i and the total number of
moles present, respectively. The mole fraction is always smaller than 1. The partial
pressure of A can now express as:
Similarly,
Note that the sum of the mole fractions for a mixture of gases must be unity.
If only two components are present, then:
If a system contains more than two gases, then the partial pressure of the ith
component is related to the total pressure by:
How are partial pressures determined? A manometer can measure only the
total pressure of a gaseous mixture. To obtain the partial pressures, the mole
fractions of the components are needed to know, which would involve elaborate
chemical analyses. The most direct method of measuring partial pressures is using
a mass spectrometer. The relative intensities of the peaks in a mass spectrum are
directly proportional to the amounts, and hence to the mole fractions, of the gases
present.
Example: A mixture of gases contains 4.46 moles of neon (Ne), 0.74 mole of argon (Ar), and
2.15 moles of xenon (Xe). Calculate the partial pressures of the gases if the total
pressure is 2.00 atm at a certain temperature.
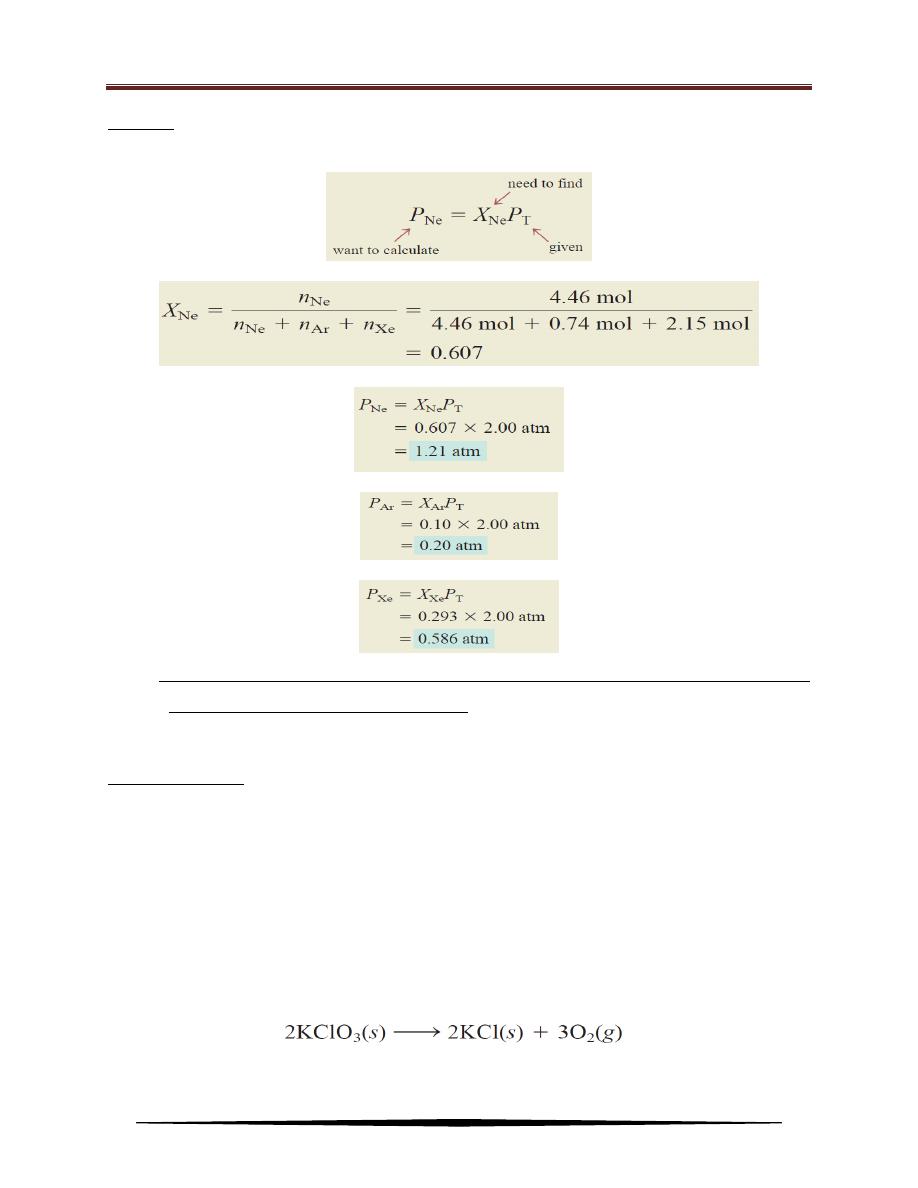
Chemistry for 1st class/Physics
Dr. Alaa Hussein Jalil
29
Solution: The partial pressure of Ne (P
Ne
) is equal to the product of its mole fraction (X
Ne
) and
the total pressure (P
T
):
The mole fraction of Ne can calculate as follows:
Therefore,
Similarly,
and
Check: Make sure that the sum of the partial pressures is equal to the given total pressure; that
is, (1.21 + 0.20 + 0.586) atm = 2.00 atm.
Practice Exercise: A sample of natural gas contains 8.24 moles of methane (CH
4
), 0.421 mole of
ethane (C
2
H
6
), and 0.116 mole of propane (C
3
H
8
). If the total pressure of
the gases is 1.37 atm, what are the partial pressures of the gases?
Dalton’s law of partial pressures is useful for calculating volumes of gases
collected over water. For example, when potassium chlorate (KClO
3
) is heated, it
decomposes to KCl and O
2
:
The oxygen gas can be collected over water, as shown in Figure as follows:
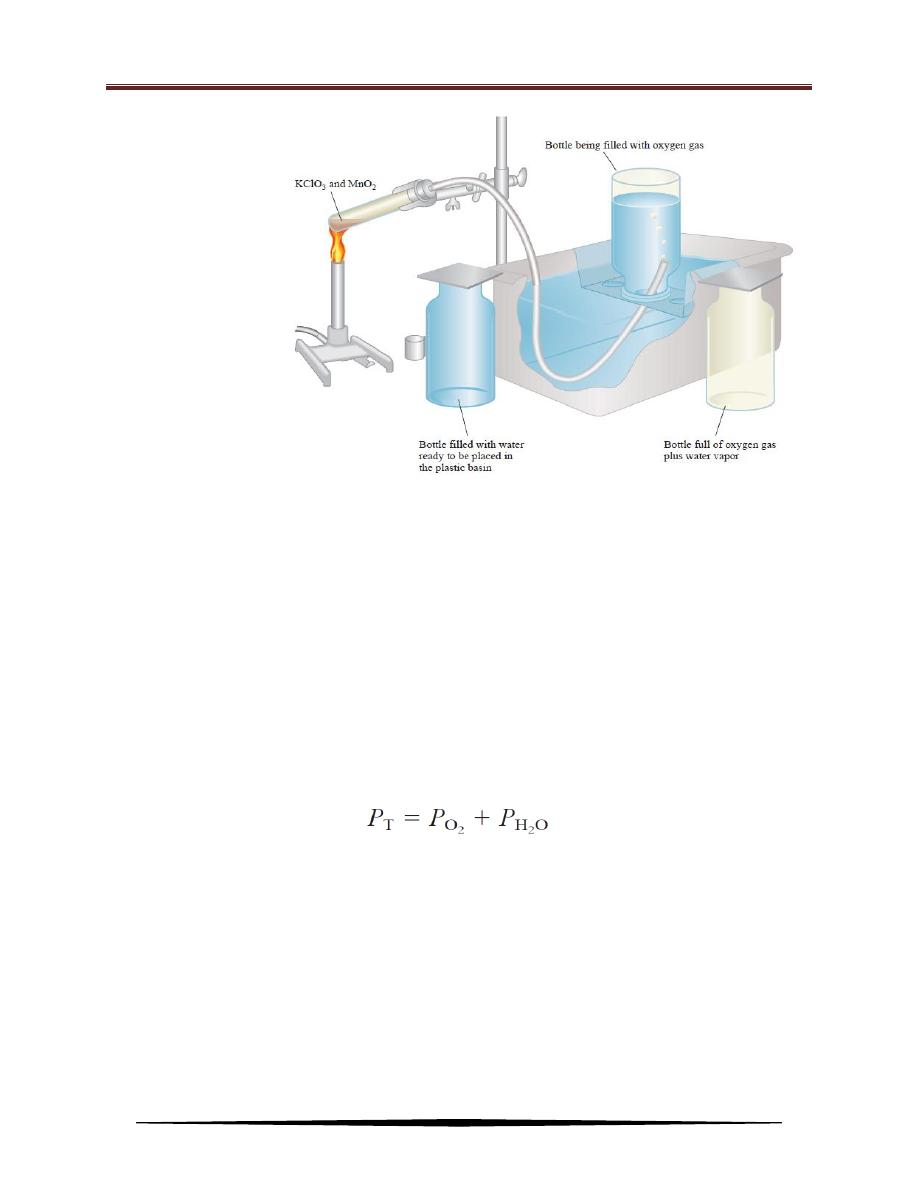
Chemistry for 1st class/Physics
Dr. Alaa Hussein Jalil
30
Initially, the inverted bottle is completely filled with water. As oxygen gas is
generated, the gas bubbles rise to the top and displace water from the bottle. This
method of collecting a gas is based on the assumptions that the gas does not react
with water and that it is not appreciably soluble in it. These assumptions are valid
for oxygen gas, but not for gases such as NH
3
, which dissolves readily in water.
The oxygen gas collected in this way is not pure, however, because water vapor is
also present in the bottle. The total gas pressure is equal to the sum of the pressures
exerted by the oxygen gas and the water vapor:
Consequently, the pressure caused by the presence of water vapor when the
amount of O
2
generated calculates must be allowed.
An
apparatus
for
collecting gas over water.
The oxygen generated by
heating potassium chlorate
(KClO
3
) in the presence of
a
small
amount
of
manganese
dioxide
(MnO
2
), which speeds up
the reaction, is bubbled
through
water
and
collected in a bottle as
shown. Water originally
present in the bottle is
pushed into the trough by
the oxygen gas.
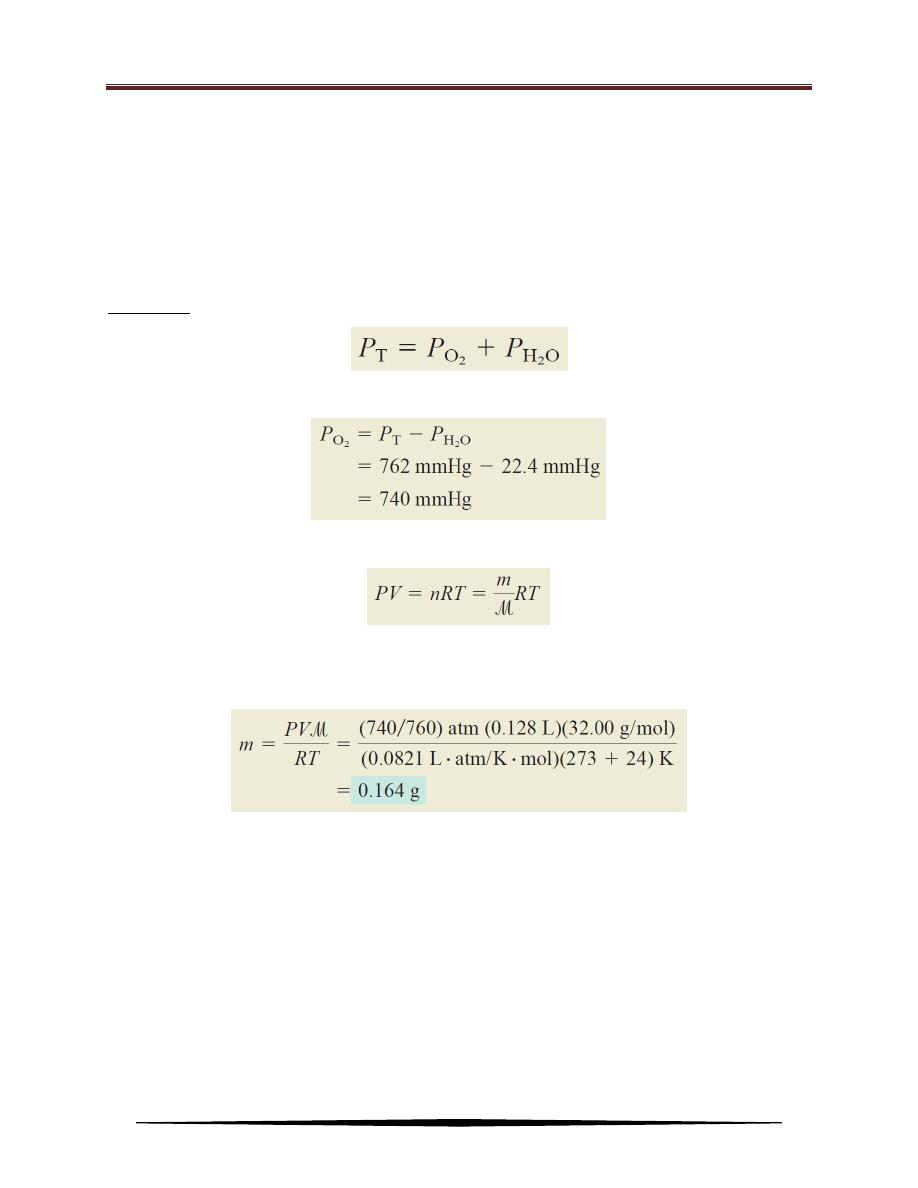
Chemistry for 1st class/Physics
Dr. Alaa Hussein Jalil
31
Example: Oxygen gas generated by the decomposition of potassium chlorate is
collected as shown in Figure above. The volume of oxygen collected at
24
o
C and atmospheric pressure of 762 mmHg is 128 mL. Calculate the
mass (in grams) of oxygen gas obtained. The pressure of the water vapor
at 24
o
C is 22.4 mmHg.
Solution: From Dalton’s law of partial pressures:
Therefore,
From the ideal gas equation:
where m and are the mass of O
2
collected and the molar mass of O
2
, respectively.
Rearranging:
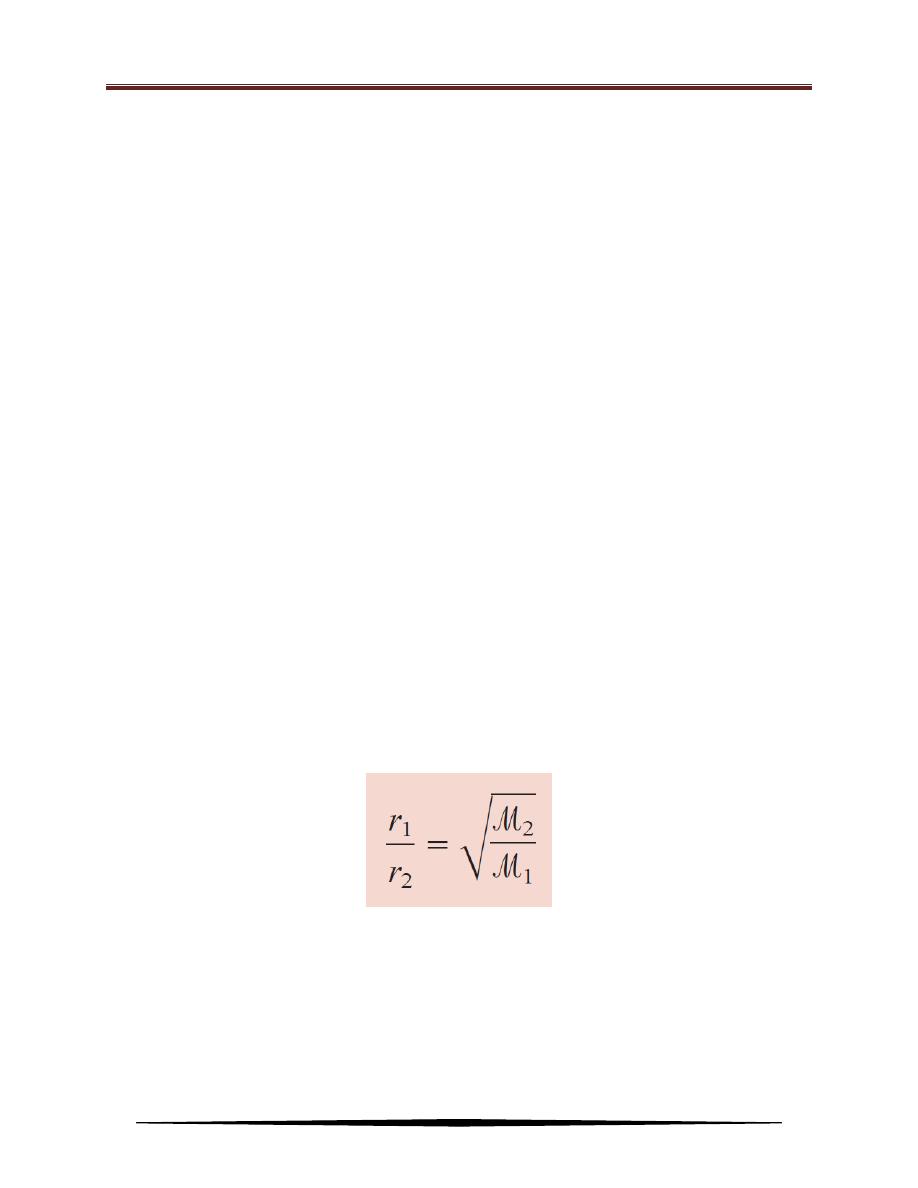
Chemistry for 1st class/Physics
Dr. Alaa Hussein Jalil
32
Gas Diffusion and Effusion
Gas Diffusion
A direct demonstration of random motion is provided by diffusion, the
gradual mixing of molecules of one gas with molecules of another by virtue of their
kinetic properties. Despite the fact that molecular speeds are very great, the
diffusion process takes a relatively long time to complete. For example, when a
bottle of concentrated ammonia solution is opened at one end of a lab bench, it
takes some time before a person at the other end of the bench can smell it. The
reason is that a molecule experiences numerous collisions while moving from one
end of the bench to the other. Thus, diffusion of gases always happens gradually,
and not instantly as molecular speeds seem to suggest. Furthermore, because the
root-mean-square speed of a light gas is greater than that of a heavier gas (refer to
example above), a lighter gas will diffuse through a certain space more quickly
than will a heavier gas.
In 1832 the Scottish chemist Thomas Graham found that under the same
conditions of temperature and pressure, rates of diffusion for gases are inversely
proportional to the square roots of their molar masses. This statement, now known
as Graham’s law of diffusion, is expressed mathematically as:
where r
1
and r
2
are the diffusion rates of gases 1 and 2, and
M
1
and
M
2
are their
molar masses, respectively.
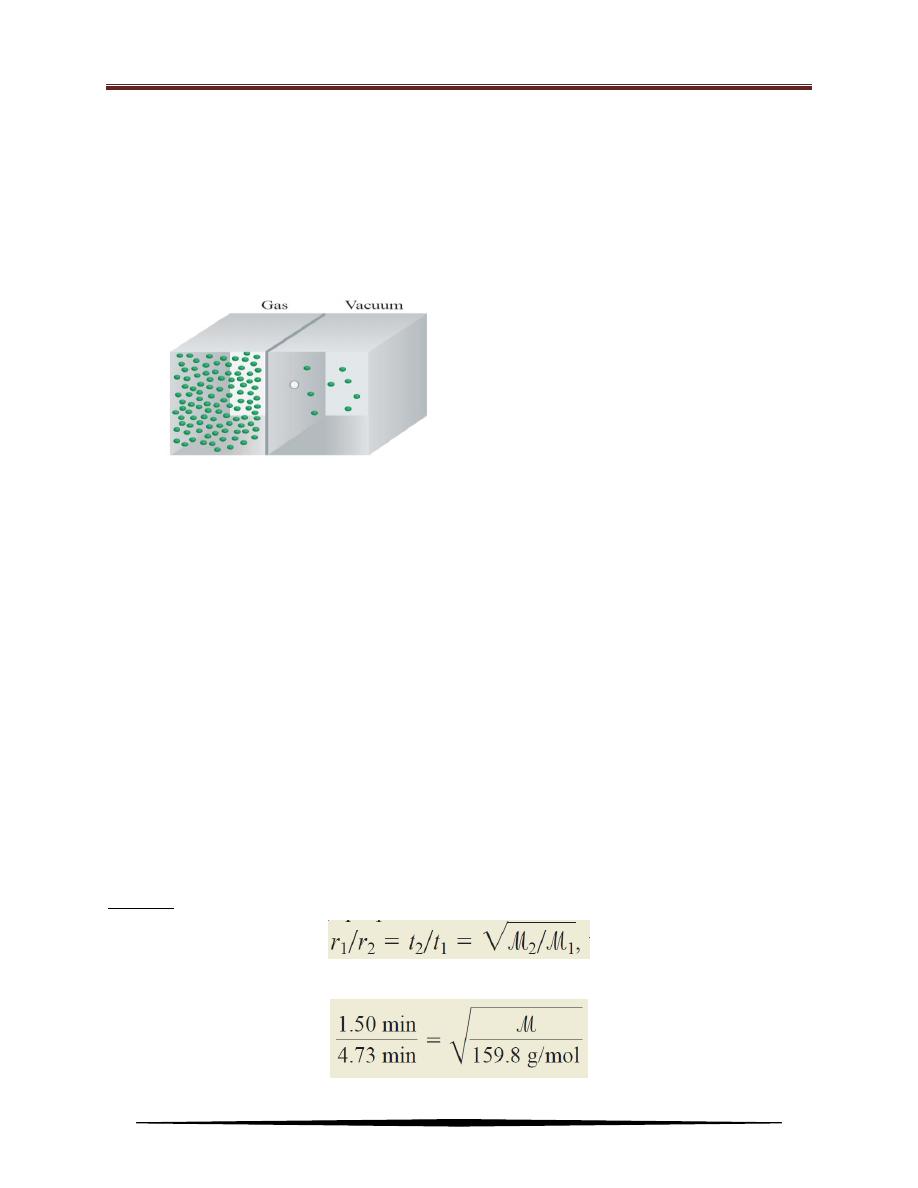
Chemistry for 1st class/Physics
Dr. Alaa Hussein Jalil
33
Gas Effusion
Whereas diffusion is a process by which one gas gradually mixes with
another, effusion is the process by which a gas under pressure escapes from one
compartment of a container to another by passing through a small opening. The
effusion of a gas into a vacuum can depict in Figure:
Although effusion differs from diffusion in nature, the rate of effusion of a
gas has the same form as Graham’s law of diffusion (see the equation above). A
helium-filled rubber balloon deflates faster than an air-filled one because the rate
of effusion through the pores of the rubber is faster for the lighter helium atoms
than for the air molecules. Industrially, gas effusion is used to separate uranium
isotopes in the forms of gaseous
235
UF
6
and
238
UF
6
. By subjecting the gases to
many stages of effusion, scientists were able to obtain highly enriched
235
U isotope,
which was used in the construction of atomic bombs during World War II.
Example: A flammable gas made up only of carbon and hydrogen is found to effuse through a
porous barrier in 1.50 min. Under the same conditions of temperature and pressure, it
takes an equal volume of bromine vapor 4.73 min to effuse through the same barrier.
Calculate the molar mass of the unknown gas, and suggest what this gas might be.
Solution: From the molar mass of Br
2
:
where t
1
and t
2
are the times for effusion for gases 1 and 2, respectively.
Gas
effusion.
Gas
molecules move from a
high-pressure region
(left) to a low-pressure
one through a pinhole.
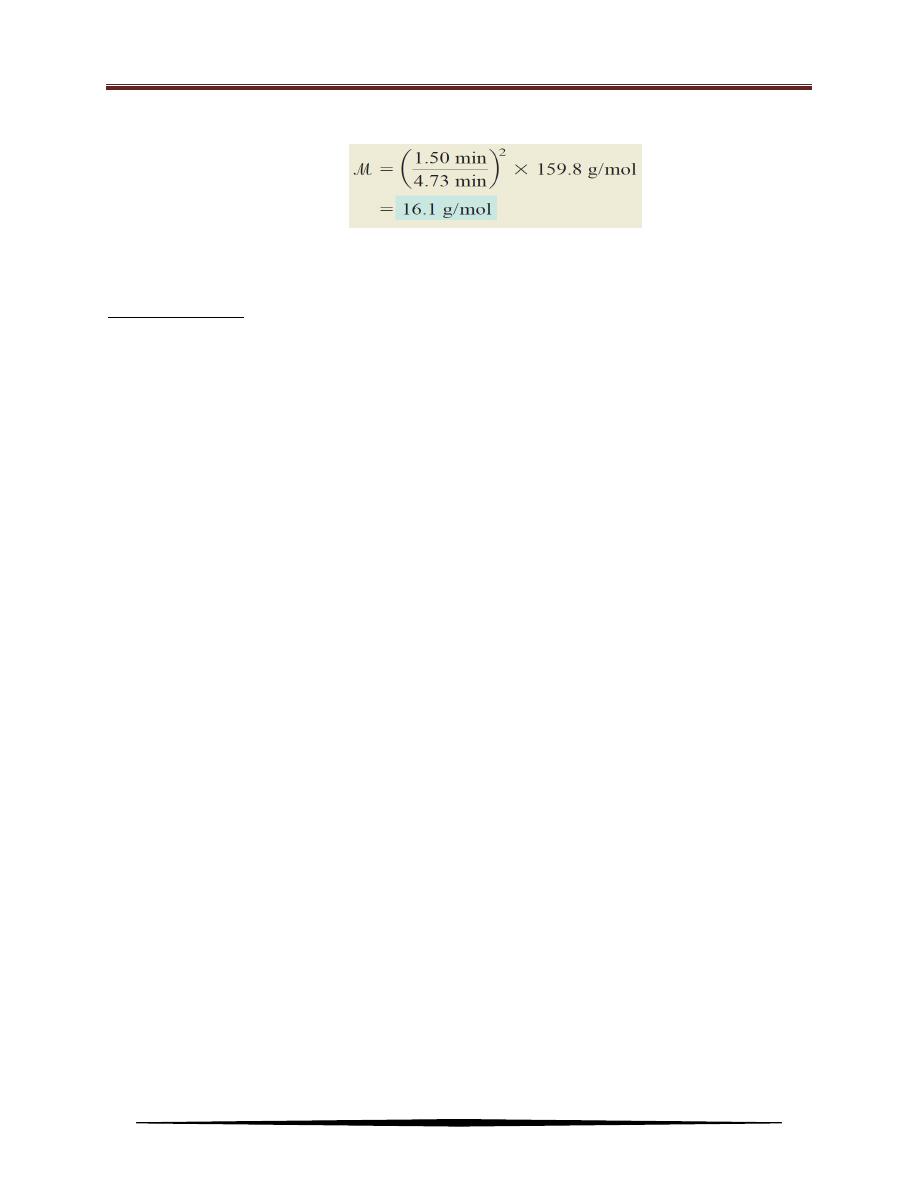
Chemistry for 1st class/Physics
Dr. Alaa Hussein Jalil
34
where
M is the molar mass of the unknown gas. Solving for M, can obtain:
Because the molar mass of carbon is 12.01 g and that of hydrogen is 1.008 g, the gas is
methane (CH
4
).
Practice Exercise: It takes 192 s for an unknown gas to effuse through a porous wall and 84 s
for the same volume of N
2
gas to effuse at the same temperature and
pressure. What is the molar mass of the unknown gas?
Deviation from Ideal Behavior
The gas laws and the kinetic molecular theory assume that molecules in the
gaseous state do not exert any force, either attractive or repulsive, on one another.
The other assumption is that the volume of the molecules is negligibly small
compared with that of the container. A gas that satisfies these two conditions is
said to exhibit ideal behavior.
Although we can assume that real gases behave like an ideal gas, we cannot
expect them to do so under all conditions. For example, without intermolecular
forces, gases could not condense to form liquids. The important question is: Under
what conditions will gases most likely exhibit nonideal behavior?
Figure below shows PV/RT plotted against P for three real gases and an ideal
gas at a given temperature. This graph provides a test of ideal gas behavior.
According to the ideal gas equation (for 1 mole of gas), PV/RT equals 1, regardless
of the actual gas pressure. (When n = 1, PV = nRT becomes PV = RT, or PV/RT =
1.) For real gases, this is true only at moderately low pressures (≤ 5 atm);
significant deviations occur as pressure increases. Attractive forces operate among
molecules at relatively short distances. At atmospheric pressure, the molecules in a
gas are far apart and the attractive forces are negligible. At high pressures, the
density of the gas increases; the molecules are much closer to one another.
Intermolecular forces can then be significant enough to affect the motion of the
molecules, and the gas will not behave ideally.
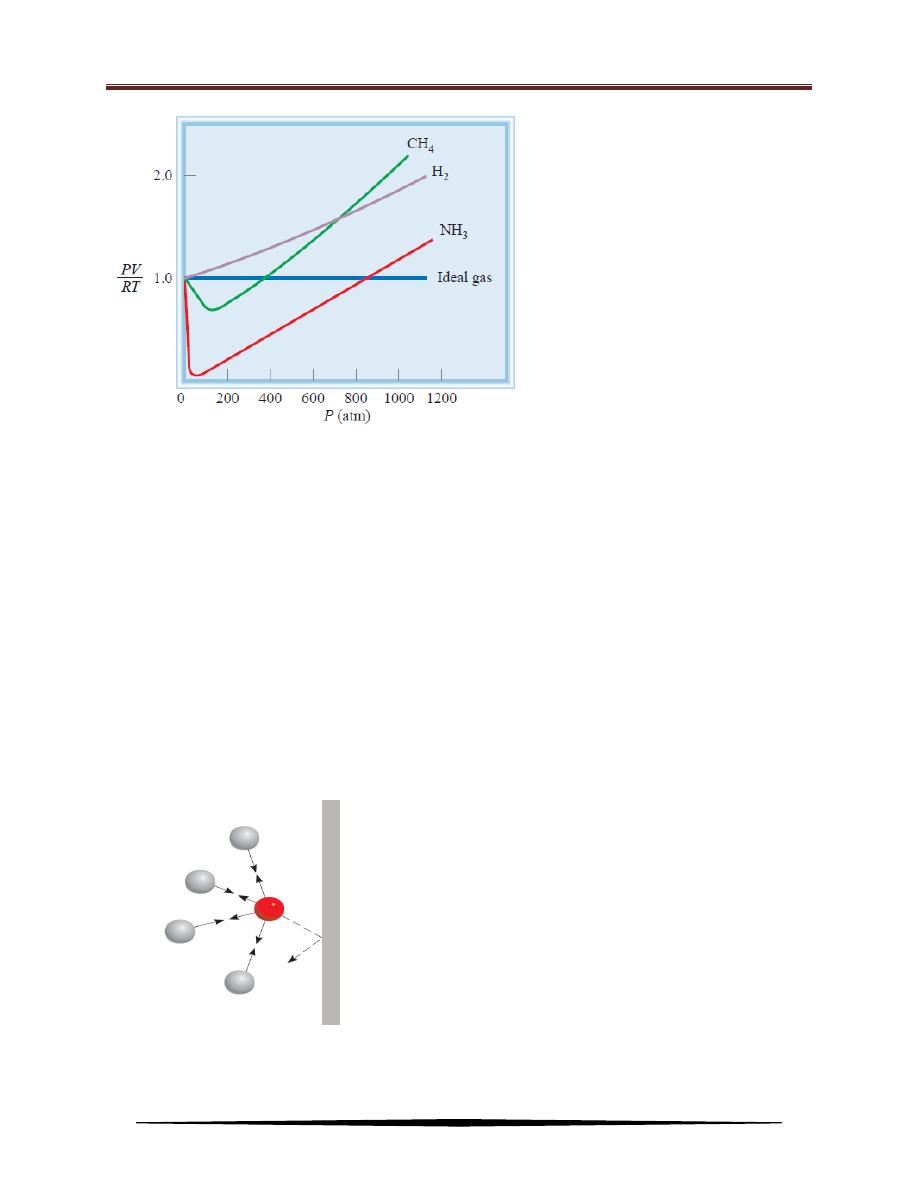
Chemistry for 1st class/Physics
Dr. Alaa Hussein Jalil
35
Another way to observe the nonideal behavior of gases is to lower the
temperature. Cooling a gas decreases the molecules’ average kinetic energy, which
in a sense deprives molecules of the drive they need to break from their mutual
attraction.
To study real gases accurately, then, we need to modify the ideal gas
equation, taking into account intermolecular forces and finite molecular volumes.
Such an analysis was first made by the Dutch physicist J. D. van der Waals in
1873. Besides being mathematically simple, van der Waals’s treatment provides us
with an interpretation of real gas behavior at the molecular level. Consider the
approach of a particular molecule toward the wall of a container:
Plot of PV_RT versus P of 1 mole
of a gas at 0
o
C. For 1 mole of an
ideal gas, PV/RT is equal to 1, no
matter what the pressure of the
gas is. For real gases, we
observe various deviations from
ideality at high pressures. At
very low pressures, all gases
exhibit ideal behavior; that is,
their PV/RT values all converge
to 1 as P approaches zero.
Effect of intermolecular forces on the
pressure exerted by a gas. The speed of a
molecule that is moving toward the
container wall (red sphere) is reduced by
the attractive forces exerted by its
neighbors (gray spheres). Consequently,
the impact this molecule makes with the
wall is not as great as it would be if no
intermolecular forces were present. In
general, the measured gas pressure is
lower than the pressure the gas would
exert if it behaved ideally.
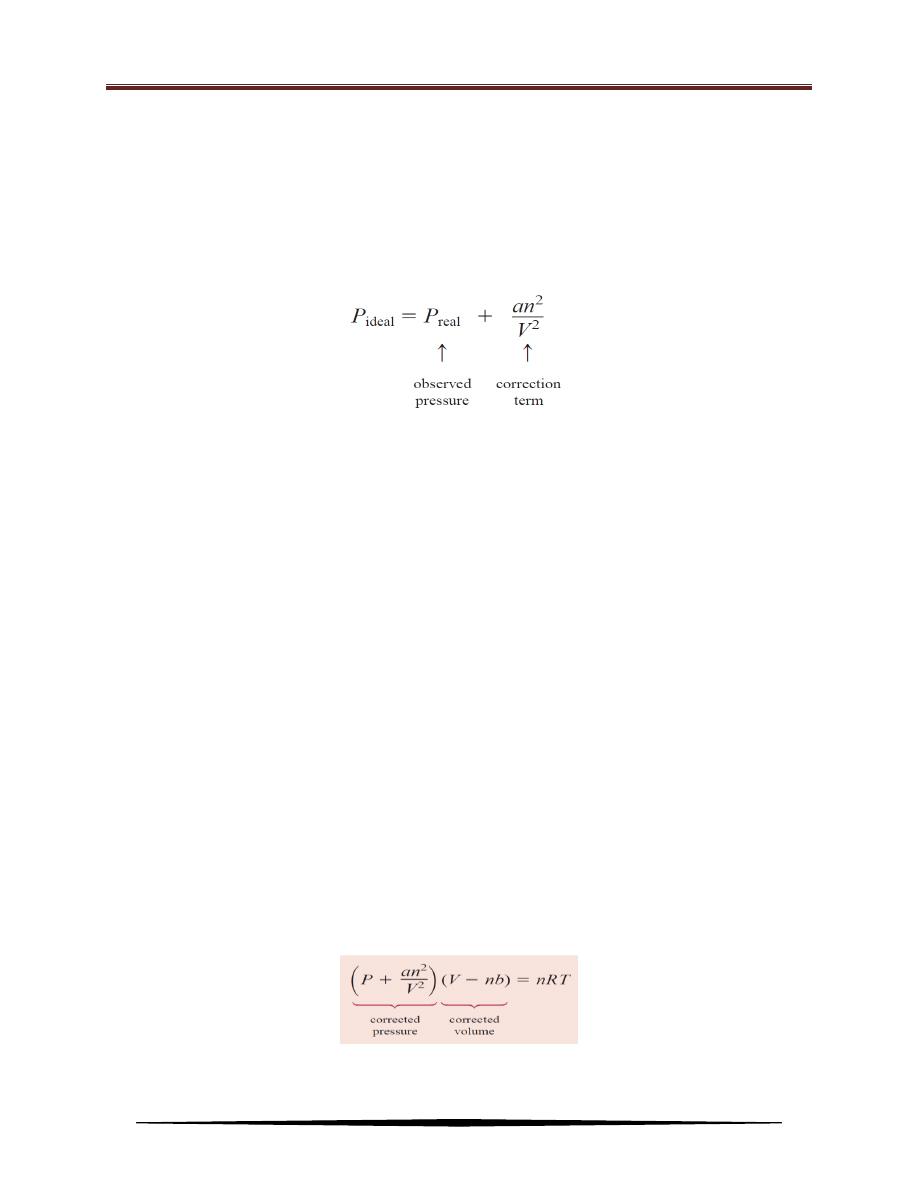
Chemistry for 1st class/Physics
Dr. Alaa Hussein Jalil
36
The intermolecular attractions exerted by its neighbors tend to soften the
impact made by this molecule against the wall. The overall effect is a lower gas
pressure than we would expect for an ideal gas. Van der Waals suggested that the
pressure exerted by an ideal gas, P
ideal
, is related to the experimentally measured;
that is, observed pressure, P
obs
, by the equation:
where a is a constant and n and V are the number of moles and volume of the gas,
respectively. The correction term for pressure (an
2
/V
2
) can be understood as
follows. The intermolecular interaction that gives rise to nonideal behavior
depends on how frequently any two molecules approach each other closely. The
number of such ―encounters‖ increases with the square of the number of molecules
per unit volume, (n/V)
2
, because the presence of each of the two molecules in a
particular region is proportional to n/V. The quantity P
ideal
is the pressure we would
measure if there were no intermolecular attractions, and so a is just a
proportionality constant.
Another correction concerns the volume occupied by the gas molecules. In
the ideal gas equation, V represents the volume of the container. However, each
molecule does occupy a finite, although small, intrinsic volume, so the effective
volume of the gas becomes (V - nb), where n is the number of moles of the gas and
b is a constant. The term nb represents the volume occupied by n moles of the gas.
Having taken into account the corrections for pressure and volume, the ideal
gas equation can rewrite as follows:
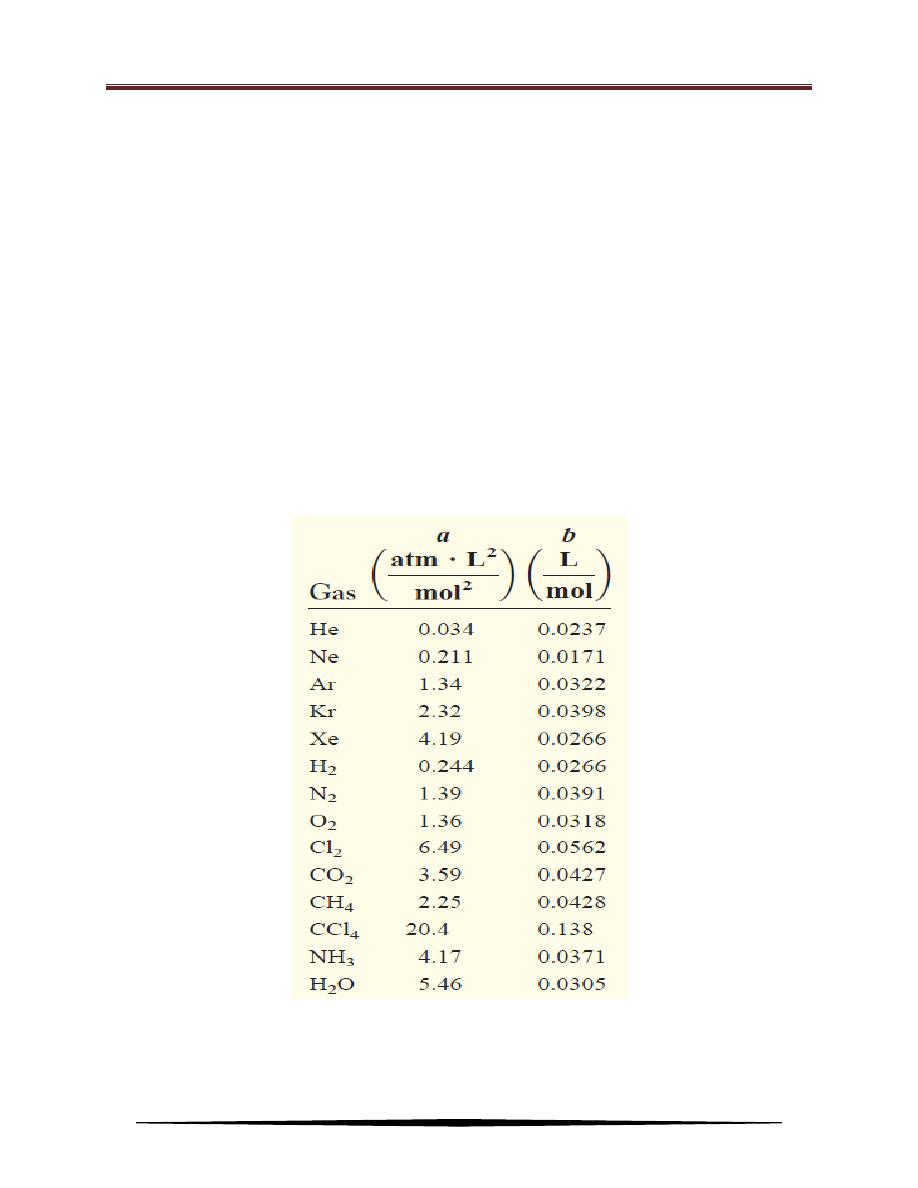
Chemistry for 1st class/Physics
Dr. Alaa Hussein Jalil
37
This equation, relating P, V, T, and n for a nonideal gas, is known as the
van der Waals equation. The van der Waals constants a and b are selected to give
the best possible agreement between above equation and observed behavior of a
particular gas.
Table below lists the values of a and b for a number of gases. The value of a
indicates how strongly molecules of a given type of gas attract one another. The
table shows that helium atoms have the weakest attraction for one another, because
helium has the smallest a value. There is also a rough correlation between
molecular size and b. Generally, the larger the molecule (or atom), the greater b is,
but the relationship between b and molecular (or atomic) size is not a simple one.
Table of van der Waals Constants of Some Common Gases
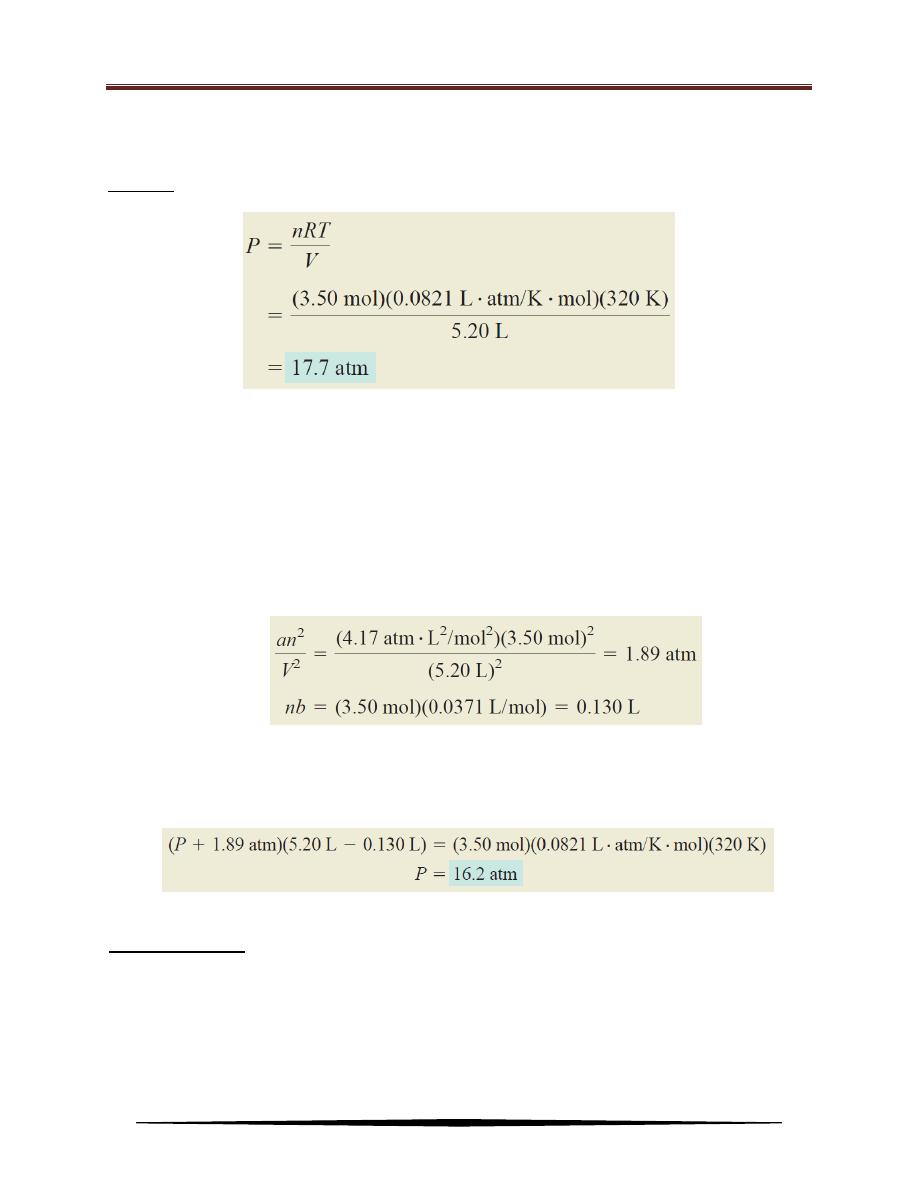
Chemistry for 1st class/Physics
Dr. Alaa Hussein Jalil
38
Example: Given that 3.50 moles of NH
3
occupy 5.20 L at 47
o
C, calculate the pressure of the gas
(in atm) using (a) the ideal gas equation and (b) the van der Waals equation.
Solution: (a) Using the ideal gas equation:
(b) By using van der Waals equation and from the Table above, we get a and b values for
NH
3
:
a = 4.17 atm . L
2
/mol
2
b= 0.0371 L/mol
so that the correction terms for pressure and volume are:
Finally, substituting these values in the van der Waals equation:
Practice Exercise: Calculate the pressure exerted by 4.37 moles of molecular chlorine confined
in a volume of 2.45 L at 38
o
C. Compare the pressure with that calculated
using the ideal gas equation. (a and b values for chlorine are:a= 6.49 atm .
L
2
/mol
2
; b= 0.0562 L/mol).
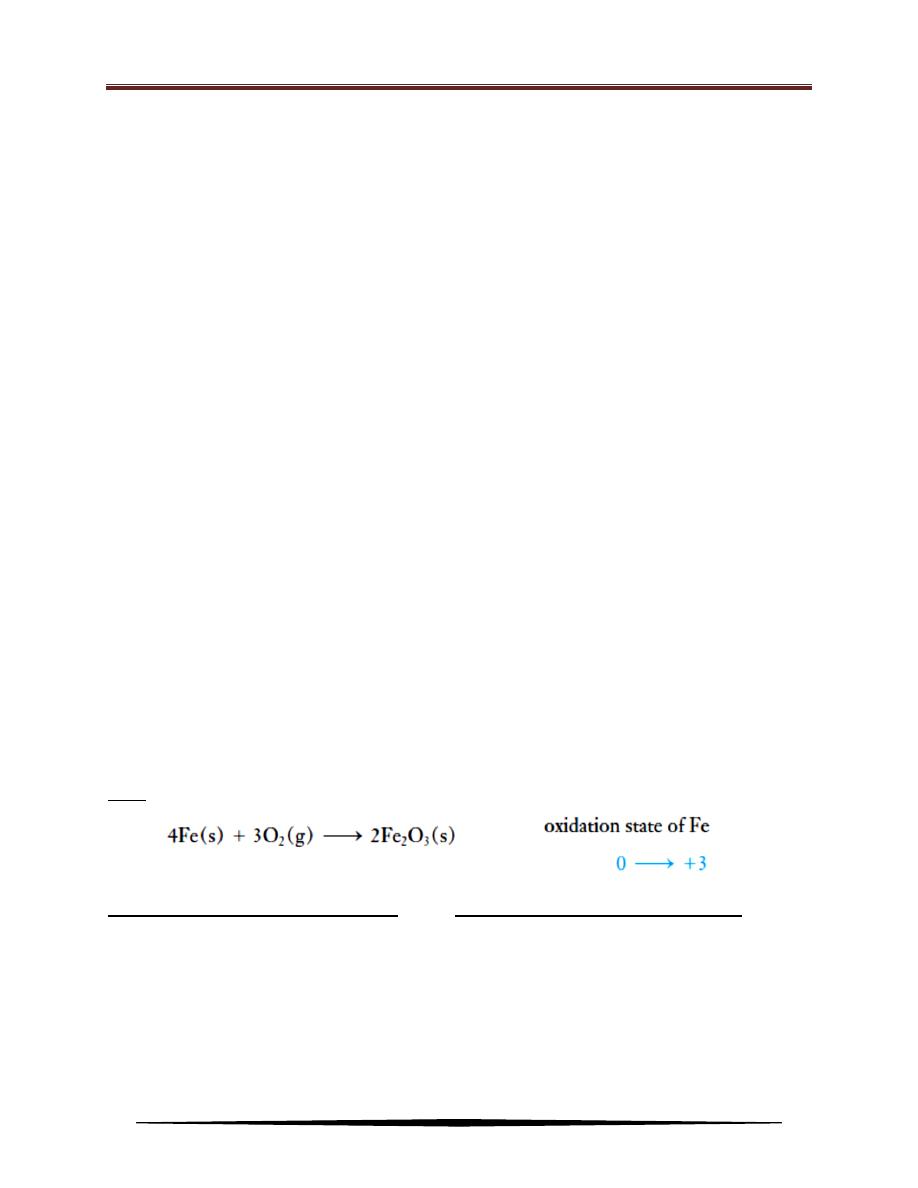
Chemistry for 1st class/Physics
Dr. Alaa Hussein Jalil
39
Types of Chemical Reactions
Chemical reactions can be classified as:
1. Oxidation–reduction reactions.
2. Combination reactions.
3. Decomposition reactions.
4. Displacement reactions.
5. Metathesis reactions.
6. Gas-formation reactions.
1. Oxidation–Reduction Reactions
The term ―oxidation‖ originally referred to the combination of a substance
with oxygen. This results in an increase in the oxidation state of an element in that
substance. Oxidation is an increase in oxidation state and corresponds to the loss,
or apparent loss, of electrons. Reduction is a decrease in oxidation state and
corresponds to a gain, or apparent gain, of electrons. Oxidation and reduction
always occur simultaneously, and to the same extent, in ordinary chemical
reactions. So they are referred to as oxidation–reduction reactions (usually we
call them redox reaction).
EX.: The formation of rust, Fe
2
O
3
, iron(III) oxide:
Oxidizing agents are species that:
Reducing agents are species that:
(1) oxidize other substances.
(1) reduce other substances.
(2) contain atoms that are reduced.
(2) contain atoms that are oxidized.
(3) gain (or appear to gain) electrons.
(3) lose (or appear to lose) electrons.
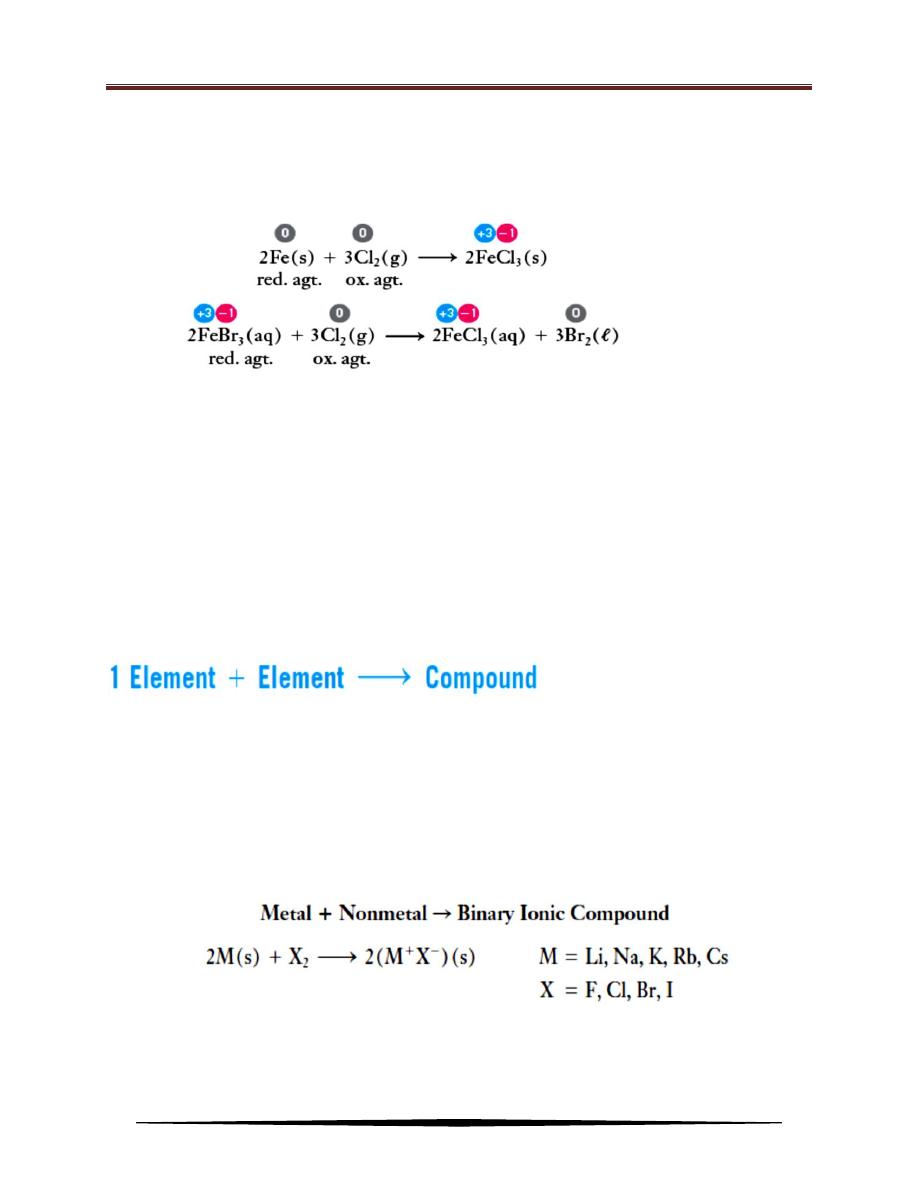
Chemistry for 1st class/Physics
Dr. Alaa Hussein Jalil
40
The following equations represent examples of redox reactions. Oxidation
states are shown above the formulas, and oxidizing and reducing agents are
indicated:
2. Combination Reactions
Reactions in which two or more substances combine to form a single
compound are called combination reactions. They may involve:
(1) The combination of two elements to form a compound.
(2) The combination of an element and a compound to form a single new
compound.
(3) The combination of two compounds to form a single new compound.
For this type of combination reaction, each element goes from an
uncombined state, where its oxidation state is zero, to a combined state in a
compound, where its oxidation state is not zero. Thus reactions of this type are
oxidation–reduction reactions. Most metals (low electronegativity) react with most
nonmetals (higher electronegativity) to form binary ionic compounds.
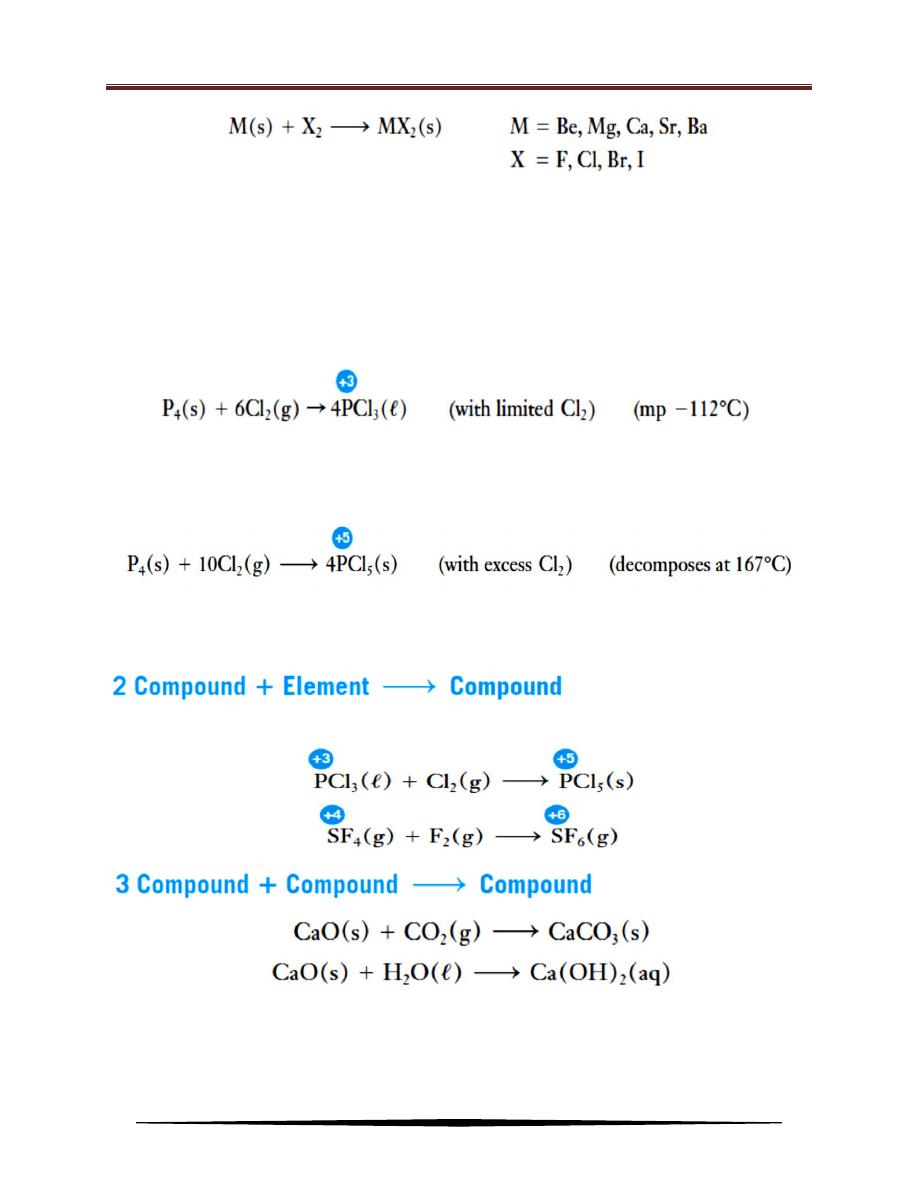
Chemistry for 1st class/Physics
Dr. Alaa Hussein Jalil
41
When two nonmetals combine with each other, they form binary covalent
compounds. In such reactions, the oxidation state of the element with the more
positive oxidation state is often variable, depending on reaction conditions. For
example, phosphorus combines with a limited amount of chlorine to form
phosphorus trichloride, in which phosphorus exhibits the +3 oxidation state.
With an excess of chlorine, the product is phosphorus pentachloride, which
contains phosphorus in the +5 oxidation state:
In general, a higher oxidation state of a nonmetal is formed when it reacts
with an excess of another more electronegative nonmetal.
Combination reactions of this type are also oxidation–reduction reactions.
3. Decomposition Reactions
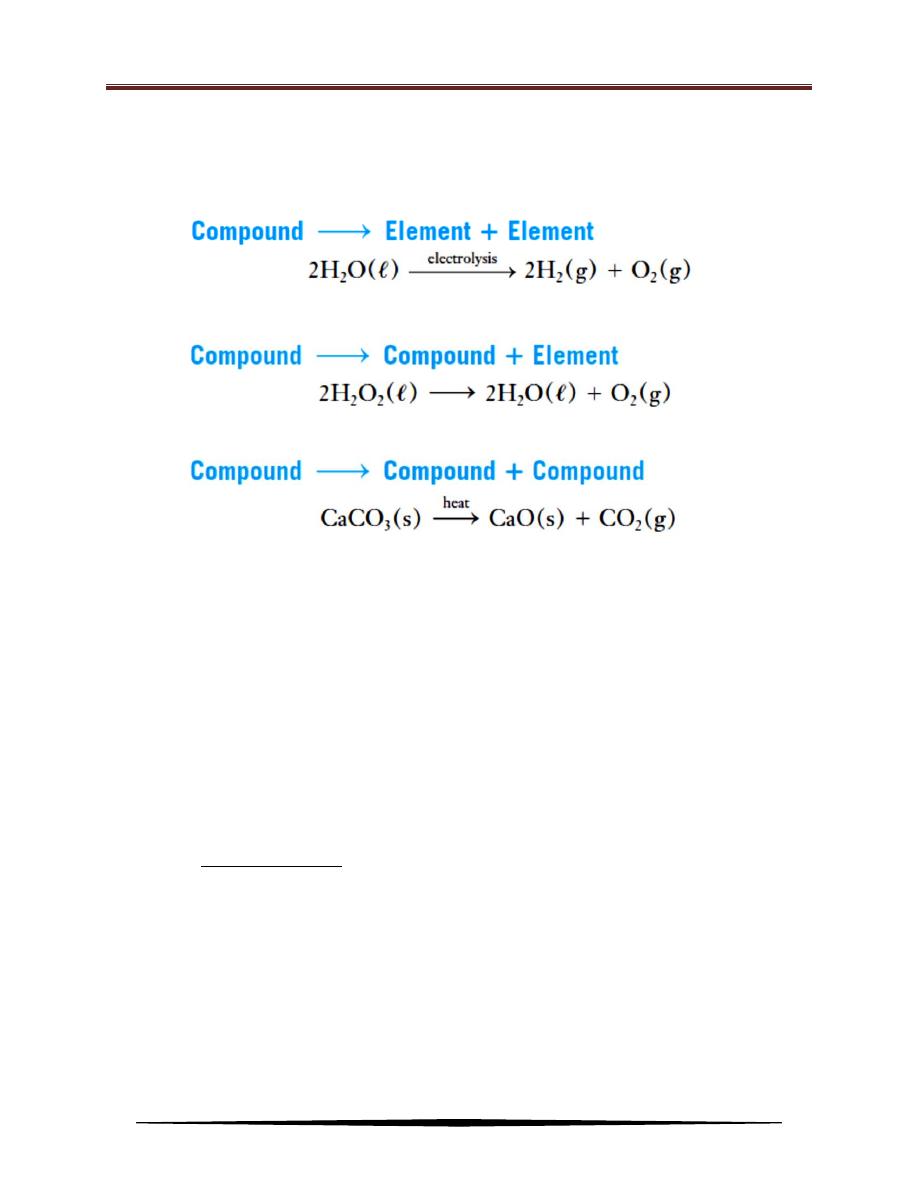
Chemistry for 1st class/Physics
Dr. Alaa Hussein Jalil
42
Decomposition reactions are those in which a compound decomposes
to produce:
(1) Two elements.
(2) One or more elements and one or more compounds.
(3) Two or more compounds.
4. Displacement Reactions
Reactions in which one element displaces another from a compound are
called displacement reactions. These reactions are always redox reactions. The
lower the electronegativity of a metal, the more readily that metal undergoes
oxidation. More active (less electronegative) metals displace less active (more
electronegative) metals or hydrogen from their compounds in aqueous solution to
form the oxidized form of the more active metal and the reduced (free metal) form
of the other metal or hydrogen gas.
The electronegativity of an element is a measure of the relative tendency of
an atom to attract electrons to itself when it is chemically combined with another
atom. Elements with high electronegativities (nonmetals) often gain electrons to
form anions. The higher the electronegativity, the more stable the anions that are
formed. While elements with low electronegativities (metals) often lose electrons
to form cations. The lower the electronegativity, the more stable the cations that
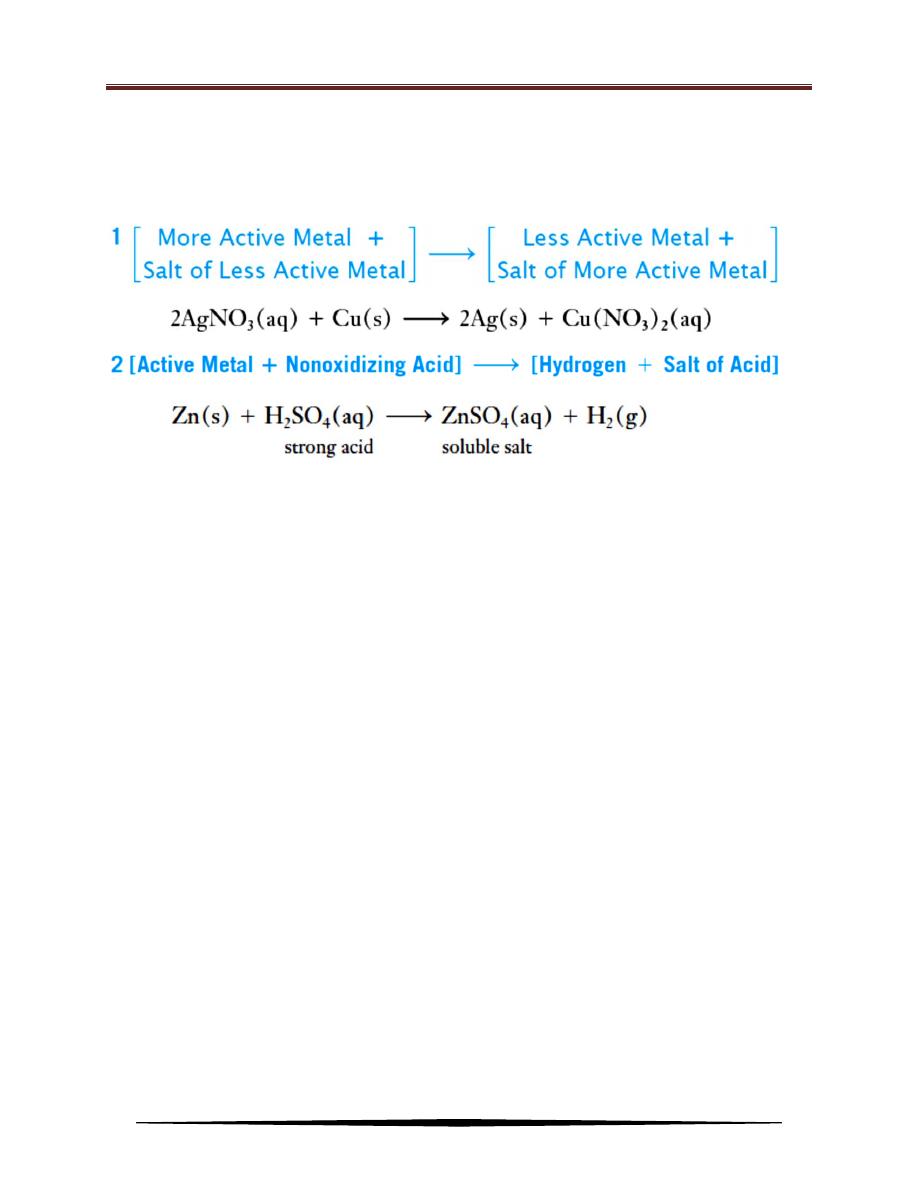
Chemistry for 1st class/Physics
Dr. Alaa Hussein Jalil
43
are formed
.
For the representative elements in the periodic table, electronegativities
usually increase from left to right across periods and decrease from top to bottom
within groups.
Activity Series of Some Elements
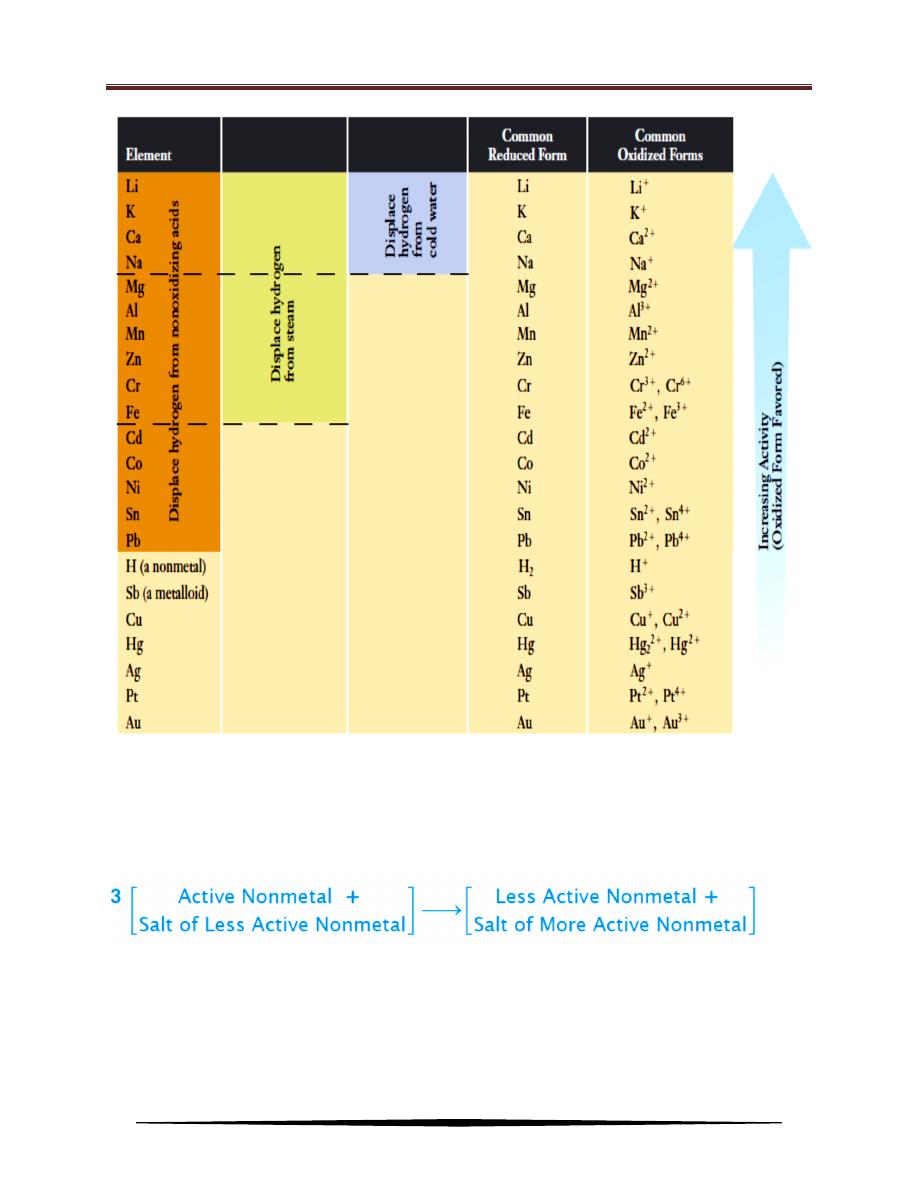
Chemistry for 1st class/Physics
Dr. Alaa Hussein Jalil
44
When any metal listed above hydrogen in this series is added to a solution of
a nonoxidizing acid such as hydrochloric acid, HCl, or sulfuric acid, H
2
SO
4
, the
metal dissolves to produce hydrogen, and a salt is formed.
Many nonmetals displace less active nonmetals from combination with a
metal or other cation.
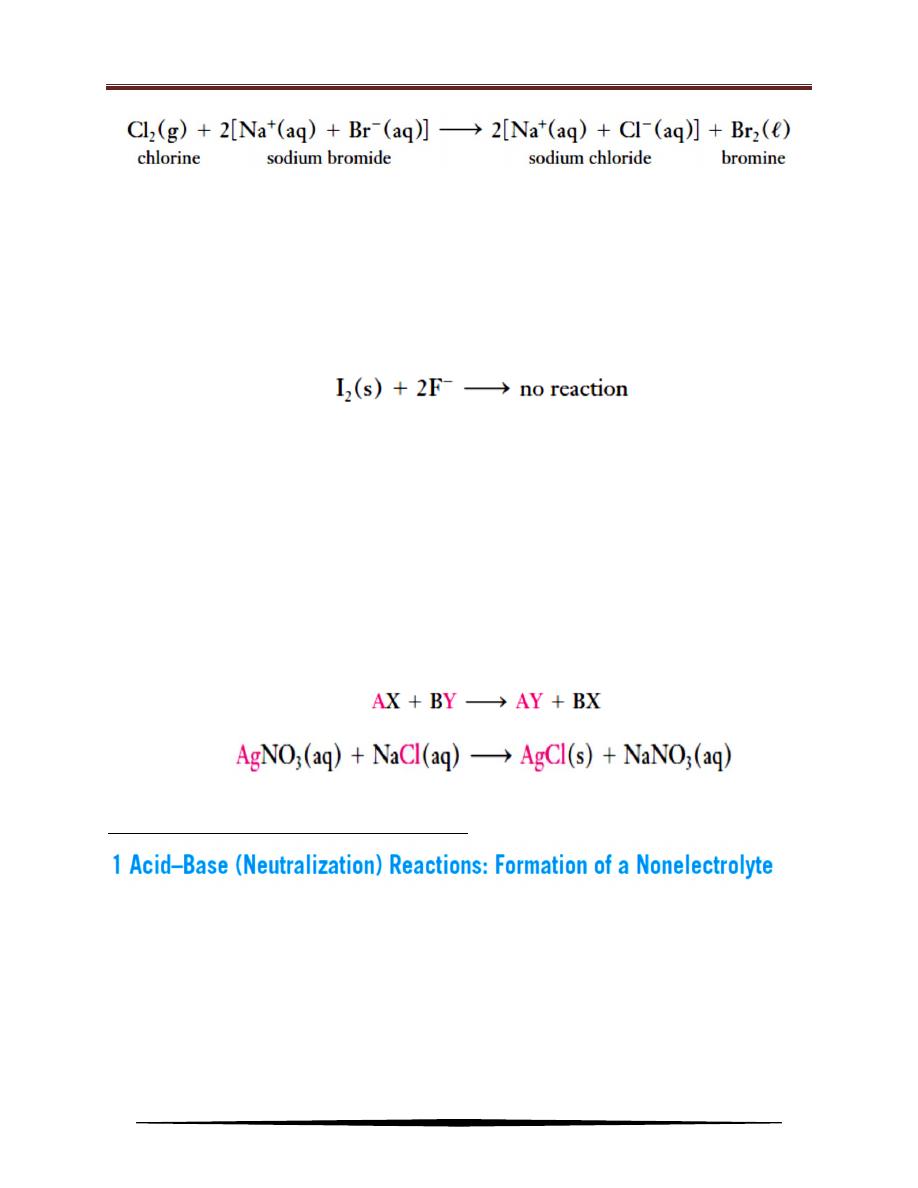
Chemistry for 1st class/Physics
Dr. Alaa Hussein Jalil
45
Each halogen will displace less active (less electronegative) halogens from
their binary salts; that is, the order of decreasing activities is:
F
2
> Cl
2
> Br
2
> I
2
Conversely, a halogen will not displace more active (more electronegative)
members from their salts:
5. Metathesis Reactions
In many reactions between two compounds in aqueous solution, the positive
and negative ions appear to ―change partners‖ to form two new compounds, with
no change in oxidation numbers. Such reactions are called metathesis reactions
(sometimes referred to as double displacement reactions).
Metathesis reactions can be classified into:
Acid–base reactions are among the most important kinds of chemical
reactions. The reaction of an acid with a metal hydroxide base produces a salt and
water. Such reactions are called neutralization reactions because the typical
properties of acids and bases are neutralized. In nearly all neutralization reactions,
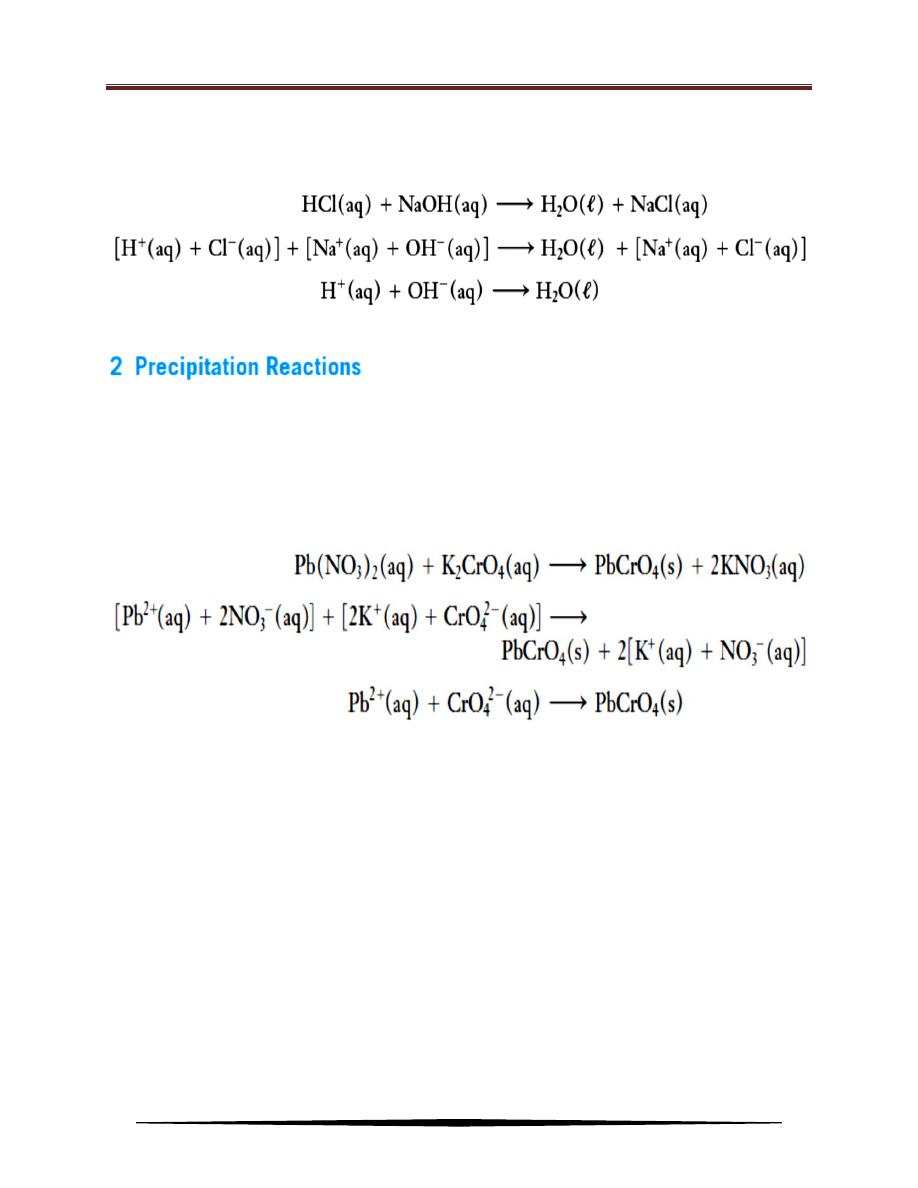
Chemistry for 1st class/Physics
Dr. Alaa Hussein Jalil
46
the driving force is the combination of H
+
(aq) from an acid and OH
¯
(aq) from a
base (or a base plus water) to form water molecules.
In precipitation reactions an insoluble solid, a precipitate, forms and then
settles out of solution. The driving force for these reactions is the strong attraction
between cations and anions. This results in the removal of ions from solution by
the formation of a precipitate.
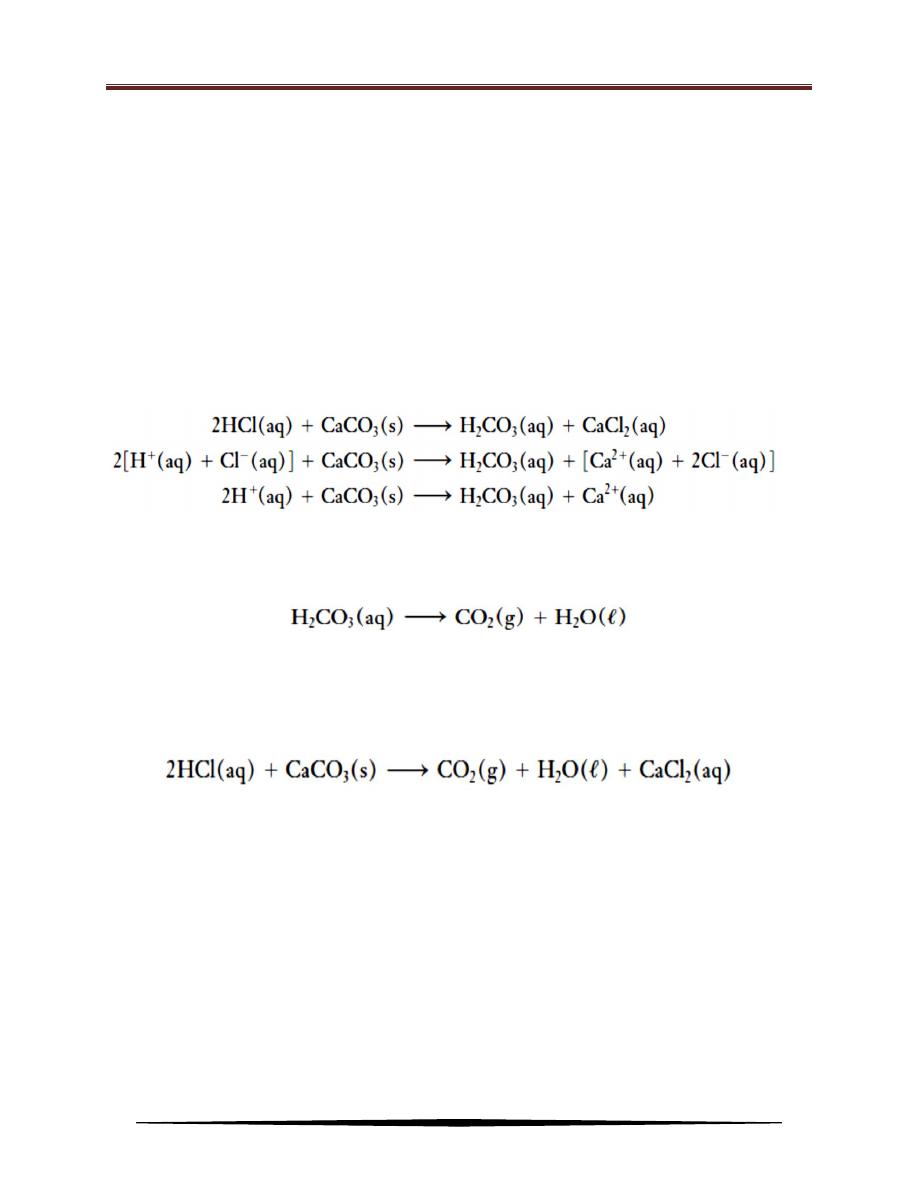
Chemistry for 1st class/Physics
Dr. Alaa Hussein Jalil
47
6. Gas-Formation Reactions
When there are no gaseous reactants, the formation of an insoluble or
slightly soluble gas provides a driving force for a type of reaction that we call a
gas-formation reaction. The only common gases that are very soluble in water are
HCl(g) and NH
3
(g). The low solubility of other gases can force a reaction to
proceed if they are formed as a reaction product.
When an acid—for example, hydrochloric acid—is added to solid calcium
carbonate, a reaction occurs in which carbonic acid, a weak acid, is produced.
The heat generated in the reaction causes thermal decomposition of carbonic
acid to gaseous carbon dioxide and water:
Most of the CO
2
bubbles off, and the reaction goes to completion (with
respect to the limiting reactant). The net effect is the conversion of ionic species
into nonionized molecules of a gas (CO
2
) and water.